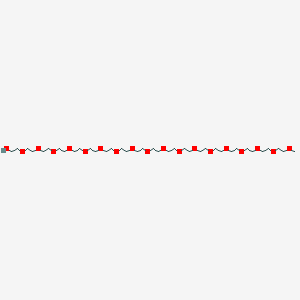
mPEG18-OH
- Click on QUICK INQUIRY to receive a quote from our team of experts.
- With the quality product at a COMPETITIVE price, you can focus more on your research.
Overview
Description
Methoxy polyethylene glycol 18-hydroxy (mPEG18-OH) is a monodispersed polyethylene glycol derivative with a molecular formula of C37H76O19 and a molecular weight of 824.99 g/mol . It is a white powder that is soluble in water and many organic solvents. This compound is widely used in various fields, including medical research, drug delivery, nanotechnology, and new materials research .
Preparation Methods
Synthetic Routes and Reaction Conditions
The synthesis of methoxy polyethylene glycol 18-hydroxy typically involves the polymerization of ethylene oxide in the presence of a methoxy initiator. The reaction is carried out under controlled conditions to achieve the desired molecular weight and polydispersity. The resulting polymer is then purified to remove any unreacted monomers and by-products .
Industrial Production Methods
In industrial settings, the production of methoxy polyethylene glycol 18-hydroxy involves large-scale polymerization reactors. The process is optimized for high yield and purity, with stringent quality control measures to ensure consistency. The final product is typically stored at low temperatures to maintain its stability .
Chemical Reactions Analysis
Types of Reactions
Methoxy polyethylene glycol 18-hydroxy can undergo various chemical reactions, including:
Oxidation: This reaction involves the conversion of the hydroxyl group to a carbonyl group using oxidizing agents.
Reduction: The reduction of the carbonyl group back to a hydroxyl group can be achieved using reducing agents.
Substitution: The hydroxyl group can be substituted with other functional groups through nucleophilic substitution reactions.
Common Reagents and Conditions
Oxidation: Common oxidizing agents include potassium permanganate and chromium trioxide.
Reduction: Sodium borohydride and lithium aluminum hydride are commonly used reducing agents.
Substitution: Reagents such as alkyl halides and acyl chlorides are used for nucleophilic substitution reactions.
Major Products Formed
The major products formed from these reactions depend on the specific reagents and conditions used. For example, oxidation of methoxy polyethylene glycol 18-hydroxy can yield aldehydes or ketones, while substitution reactions can produce various ether or ester derivatives .
Scientific Research Applications
Methoxy polyethylene glycol 18-hydroxy has a wide range of applications in scientific research:
Mechanism of Action
The mechanism of action of methoxy polyethylene glycol 18-hydroxy involves its ability to modify the surface properties of materials. By attaching to surfaces, it can enhance hydrophilicity, reduce protein adsorption, and improve biocompatibility. These effects are mediated through interactions with molecular targets such as cell membranes and extracellular matrix proteins .
Comparison with Similar Compounds
Similar Compounds
- Methoxy polyethylene glycol 12-hydroxy (mPEG12-OH)
- Methoxy polyethylene glycol 24-hydroxy (mPEG24-OH)
- Methoxy polyethylene glycol 45-hydroxy (mPEG45-OH)
Uniqueness
Methoxy polyethylene glycol 18-hydroxy is unique due to its specific molecular weight and chain length, which provide an optimal balance between solubility and functionality. Compared to shorter or longer polyethylene glycol derivatives, it offers superior performance in drug delivery and surface modification applications .
Biological Activity
Introduction
mPEG18-OH, or methoxy polyethylene glycol with an 18-unit ethylene glycol chain, is a polymer widely used in biopharmaceuticals and drug delivery systems due to its unique properties. Its biological activity is primarily attributed to its hydrophilicity, biocompatibility, and ability to modify the pharmacokinetics of therapeutic agents. This article explores the biological activity of this compound, highlighting its interactions with proteins, effects on immune responses, and implications for drug delivery.
Hydrophilicity and Biocompatibility
This compound exhibits significant hydrophilic properties, which enhance its solubility in aqueous environments. This property is crucial for drug formulation, as it improves the stability and bioavailability of hydrophobic drugs. The biocompatibility of this compound minimizes adverse immune responses when used in vivo, making it an ideal candidate for medical applications.
Mechanism of Action
The biological activity of this compound can be attributed to several mechanisms:
- Protein Interaction : mPEG chains can interact with proteins through hydrogen bonding and van der Waals forces. This interaction can alter protein conformation and stability, impacting their biological functions.
- Immune Modulation : Studies have shown that mPEG-modified proteins can induce higher titers of anti-PEG antibodies compared to unmodified proteins. This immune response can influence the pharmacokinetics of PEGylated drugs .
- Stealth Effect : The presence of mPEG on drug molecules can create a "stealth" effect, allowing them to evade detection by the immune system, thereby prolonging circulation time in the bloodstream.
Interaction with Antibodies
Recent studies have focused on the interaction between this compound and specific antibodies. For instance, site-directed mutagenesis experiments demonstrated that certain aromatic residues in antibodies play a critical role in binding to mPEG chains. Mutations that disrupted these interactions significantly reduced binding affinity .
Table 1: Critical Residues for mPEG Interaction
Residue | Mutation Type | Binding Activity |
---|---|---|
Y32 H | Tyrosine | Similar |
W33 H | Phenylalanine | Reduced |
R101 H | Alanine | Abolished |
This table summarizes key findings regarding how specific mutations affect the binding activity of antibodies to this compound.
Case Studies on Drug Delivery
Several case studies have highlighted the efficacy of this compound in drug delivery systems:
- Case Study 1: Anticancer Drug Delivery
- Researchers developed a PEGylated formulation of doxorubicin using this compound. The study found that this formulation improved therapeutic efficacy while reducing systemic toxicity compared to conventional doxorubicin treatments.
- Case Study 2: Vaccine Development
- A study investigated the use of mPEG-modified antigens in vaccine formulations. Results indicated enhanced immunogenicity and prolonged antigen presentation due to the stealth properties conferred by this compound.
Pharmacokinetics and Biodistribution
The incorporation of this compound into drug formulations has been shown to significantly alter pharmacokinetic profiles. For instance, PEGylation often results in:
- Increased Circulation Time : Drugs modified with mPEG exhibit longer half-lives in circulation due to reduced renal clearance.
- Targeted Delivery : By modifying surface characteristics, mPEG can facilitate targeted delivery to specific tissues or cells.
Properties
CAS No. |
171286-86-5 |
---|---|
Molecular Formula |
C37H76O19 |
Molecular Weight |
825.0 g/mol |
IUPAC Name |
2-[2-[2-[2-[2-[2-[2-[2-[2-[2-[2-[2-[2-[2-[2-[2-[2-(2-methoxyethoxy)ethoxy]ethoxy]ethoxy]ethoxy]ethoxy]ethoxy]ethoxy]ethoxy]ethoxy]ethoxy]ethoxy]ethoxy]ethoxy]ethoxy]ethoxy]ethoxy]ethanol |
InChI |
InChI=1S/C37H76O19/c1-39-4-5-41-8-9-43-12-13-45-16-17-47-20-21-49-24-25-51-28-29-53-32-33-55-36-37-56-35-34-54-31-30-52-27-26-50-23-22-48-19-18-46-15-14-44-11-10-42-7-6-40-3-2-38/h38H,2-37H2,1H3 |
InChI Key |
UCMLBGMTMUQKFK-UHFFFAOYSA-N |
Canonical SMILES |
COCCOCCOCCOCCOCCOCCOCCOCCOCCOCCOCCOCCOCCOCCOCCOCCOCCOCCO |
Origin of Product |
United States |
Disclaimer and Information on In-Vitro Research Products
Please be aware that all articles and product information presented on BenchChem are intended solely for informational purposes. The products available for purchase on BenchChem are specifically designed for in-vitro studies, which are conducted outside of living organisms. In-vitro studies, derived from the Latin term "in glass," involve experiments performed in controlled laboratory settings using cells or tissues. It is important to note that these products are not categorized as medicines or drugs, and they have not received approval from the FDA for the prevention, treatment, or cure of any medical condition, ailment, or disease. We must emphasize that any form of bodily introduction of these products into humans or animals is strictly prohibited by law. It is essential to adhere to these guidelines to ensure compliance with legal and ethical standards in research and experimentation.