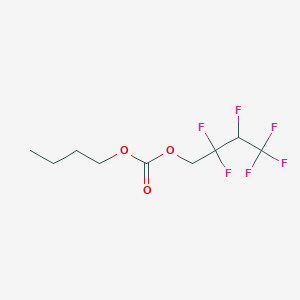
Butyl 2,2,3,4,4,4-hexafluorobutyl carbonate
- Click on QUICK INQUIRY to receive a quote from our team of experts.
- With the quality product at a COMPETITIVE price, you can focus more on your research.
Overview
Description
Butyl 2,2,3,4,4,4-hexafluorobutyl carbonate: is an organofluorine compound with the molecular formula C9H12F6O3. This compound is known for its unique properties due to the presence of multiple fluorine atoms, which impart high thermal stability and resistance to chemical reactions. It is used in various industrial applications, particularly in the field of materials science and polymer chemistry .
Preparation Methods
Synthetic Routes and Reaction Conditions: The synthesis of Butyl 2,2,3,4,4,4-hexafluorobutyl carbonate typically involves the reaction of butanol with 2,2,3,4,4,4-hexafluorobutanol in the presence of a carbonate source. The reaction is usually carried out under anhydrous conditions to prevent hydrolysis of the carbonate ester. Common reagents include phosgene or dimethyl carbonate as the carbonate source .
Industrial Production Methods: In industrial settings, the production of this compound is scaled up using continuous flow reactors to ensure consistent product quality and yield. The reaction conditions are optimized to maximize the conversion rate and minimize by-products. The final product is purified through distillation or recrystallization .
Chemical Reactions Analysis
Types of Reactions: Butyl 2,2,3,4,4,4-hexafluorobutyl carbonate undergoes various chemical reactions, including:
Hydrolysis: In the presence of water, it can hydrolyze to form butanol and 2,2,3,4,4,4-hexafluorobutanol.
Substitution: It can undergo nucleophilic substitution reactions where the carbonate group is replaced by other nucleophiles.
Oxidation and Reduction: While less common, it can participate in oxidation and reduction reactions under specific conditions.
Common Reagents and Conditions:
Hydrolysis: Acidic or basic conditions can catalyze the hydrolysis reaction.
Substitution: Nucleophiles such as amines or thiols can be used in substitution reactions.
Oxidation and Reduction: Strong oxidizing or reducing agents are required for these reactions.
Major Products:
Hydrolysis: Butanol and 2,2,3,4,4,4-hexafluorobutanol.
Substitution: Products depend on the nucleophile used.
Oxidation and Reduction: Various oxidized or reduced forms of the compound.
Scientific Research Applications
Chemistry: Butyl 2,2,3,4,4,4-hexafluorobutyl carbonate is used as a building block in the synthesis of fluorinated polymers and copolymers. Its unique properties enhance the thermal stability and chemical resistance of the resulting materials .
Biology and Medicine: In biological research, this compound is used to modify surfaces of biomaterials to improve their biocompatibility and resistance to biofouling. It is also investigated for its potential use in drug delivery systems due to its stability and low toxicity .
Industry: In industrial applications, this compound is used as a specialty solvent and in the production of high-performance coatings and adhesives. Its resistance to harsh chemical environments makes it suitable for use in extreme conditions .
Mechanism of Action
The mechanism of action of Butyl 2,2,3,4,4,4-hexafluorobutyl carbonate involves its interaction with various molecular targets depending on the application. In polymer chemistry, it acts as a monomer that can be polymerized to form high-performance materials. In biological applications, it modifies surface properties to enhance biocompatibility and reduce biofouling .
Comparison with Similar Compounds
- 2,2,3,4,4,4-Hexafluorobutyl methacrylate
- 2,2,3,3,4,4,4-Heptafluorobutyl methacrylate
- 2,2,3,4,4,4-Hexafluorobutyl acrylate
Comparison: Butyl 2,2,3,4,4,4-hexafluorobutyl carbonate is unique due to its carbonate ester group, which imparts different reactivity compared to methacrylate or acrylate esters. This difference in functional groups affects the polymerization behavior and the properties of the resulting polymers. The presence of multiple fluorine atoms in all these compounds provides high thermal stability and chemical resistance, but the specific properties and applications can vary based on the functional groups attached .
Properties
Molecular Formula |
C9H12F6O3 |
---|---|
Molecular Weight |
282.18 g/mol |
IUPAC Name |
butyl 2,2,3,4,4,4-hexafluorobutyl carbonate |
InChI |
InChI=1S/C9H12F6O3/c1-2-3-4-17-7(16)18-5-8(11,12)6(10)9(13,14)15/h6H,2-5H2,1H3 |
InChI Key |
QICOCLNMODAUSR-UHFFFAOYSA-N |
Canonical SMILES |
CCCCOC(=O)OCC(C(C(F)(F)F)F)(F)F |
Origin of Product |
United States |
Disclaimer and Information on In-Vitro Research Products
Please be aware that all articles and product information presented on BenchChem are intended solely for informational purposes. The products available for purchase on BenchChem are specifically designed for in-vitro studies, which are conducted outside of living organisms. In-vitro studies, derived from the Latin term "in glass," involve experiments performed in controlled laboratory settings using cells or tissues. It is important to note that these products are not categorized as medicines or drugs, and they have not received approval from the FDA for the prevention, treatment, or cure of any medical condition, ailment, or disease. We must emphasize that any form of bodily introduction of these products into humans or animals is strictly prohibited by law. It is essential to adhere to these guidelines to ensure compliance with legal and ethical standards in research and experimentation.