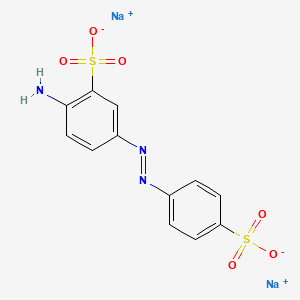
Fast Yellow AB
Overview
Description
Fast Yellow AB is an azo dye, known chemically as 2-amino-5-[(E)-(4-sulfophenyl)diazenyl]benzenesulfonic acid . It was previously used as a food dye and designated in Europe by the E number E105. it has been delisted in both Europe and the United States due to its harmful effects, including implications in non-atopic asthma .
Mechanism of Action
Fast Yellow AB, also known as 2-amino-5-[(E)-(4-sulfophenyl)diazenyl]benzenesulfonic acid, is an azo dye that was formerly used as a food dye . This article will delve into the various aspects of its mechanism of action.
Target of Action
As an azo dye, it is known to interact with various biological systems, potentially affecting cellular processes .
Biochemical Pathways
Azo dyes in general can interfere with various biochemical processes, potentially leading to alterations in cellular function .
Pharmacokinetics
As a synthetic compound, its bioavailability and pharmacokinetics would depend on various factors, including its chemical structure, the route of administration, and the individual’s metabolic processes .
Result of Action
this compound has been shown to be harmful based on toxicological data . It has been implicated in non-atopic asthma, indicating that it may have adverse effects on respiratory health .
Action Environment
The action, efficacy, and stability of this compound can be influenced by various environmental factors. These may include the pH of the environment, the presence of other compounds, and the temperature . .
Biochemical Analysis
Biochemical Properties
Fast Yellow AB plays a significant role in biochemical reactions, particularly as a biological stain. It interacts with various enzymes, proteins, and other biomolecules. The compound’s azo group (-N=N-) is known to form stable complexes with proteins, which can be utilized in staining techniques to highlight specific cellular components . This compound also interacts with enzymes involved in metabolic pathways, such as those responsible for the reduction of azo bonds, leading to the formation of aromatic amines .
Cellular Effects
This compound has been shown to affect various types of cells and cellular processes. It can influence cell function by altering cell signaling pathways, gene expression, and cellular metabolism. Studies have indicated that this compound can induce oxidative stress in cells, leading to changes in gene expression and activation of stress response pathways . Additionally, the compound’s interaction with cellular proteins can disrupt normal cellular functions, potentially leading to cytotoxic effects .
Molecular Mechanism
The molecular mechanism of this compound involves its interaction with biomolecules at the molecular level. The compound’s azo group can undergo reduction by cellular enzymes, resulting in the formation of aromatic amines, which can bind to DNA and proteins . This binding can lead to enzyme inhibition or activation, as well as changes in gene expression. This compound has also been shown to interact with cellular membranes, potentially altering membrane fluidity and function .
Temporal Effects in Laboratory Settings
In laboratory settings, the effects of this compound can change over time. The compound’s stability and degradation are important factors to consider. This compound is relatively stable under standard laboratory conditions, but it can degrade over time, especially when exposed to light and heat . Long-term exposure to this compound in in vitro or in vivo studies has shown that it can have lasting effects on cellular function, including persistent changes in gene expression and cellular metabolism .
Dosage Effects in Animal Models
The effects of this compound vary with different dosages in animal models. At low doses, the compound may have minimal effects, but at higher doses, it can induce toxic or adverse effects. Studies have shown that high doses of this compound can lead to significant cytotoxicity, oxidative stress, and disruption of normal cellular functions . Threshold effects have been observed, where a certain dosage level must be reached before noticeable effects occur .
Metabolic Pathways
This compound is involved in various metabolic pathways, particularly those related to the reduction of azo bonds. Enzymes such as azoreductases play a crucial role in the metabolism of this compound, leading to the formation of aromatic amines . These metabolic products can further interact with other enzymes and cofactors, affecting metabolic flux and metabolite levels within the cell .
Transport and Distribution
The transport and distribution of this compound within cells and tissues involve various transporters and binding proteins. The compound can be taken up by cells through active transport mechanisms and distributed within different cellular compartments . This compound can also bind to specific proteins, influencing its localization and accumulation within the cell .
Subcellular Localization
This compound’s subcellular localization is influenced by its chemical properties and interactions with cellular components. The compound can be found in various subcellular compartments, including the cytoplasm and nucleus . Post-translational modifications and targeting signals may direct this compound to specific organelles, affecting its activity and function within the cell .
Preparation Methods
Fast Yellow AB can be synthesized through a diazotization reaction followed by coupling. The synthetic route involves the diazotization of 4-aminobenzenesulfonic acid, which is then coupled with 2-aminobenzenesulfonic acid under acidic conditions . Industrial production methods typically involve large-scale batch processes where the reaction conditions are carefully controlled to ensure high yield and purity.
Chemical Reactions Analysis
Fast Yellow AB undergoes various chemical reactions, including:
Oxidation: this compound can be oxidized under specific conditions, leading to the formation of different oxidation products.
Reduction: The azo group in this compound can be reduced to form corresponding amines.
Substitution: The sulfonic acid groups in this compound can participate in substitution reactions, leading to the formation of various derivatives.
Common reagents used in these reactions include oxidizing agents like hydrogen peroxide, reducing agents like sodium dithionite, and various nucleophiles for substitution reactions . The major products formed depend on the specific reaction conditions and reagents used.
Scientific Research Applications
Fast Yellow AB has several scientific research applications:
Medicine: Although not commonly used in medicine, its derivatives and related compounds are studied for potential therapeutic applications.
Comparison with Similar Compounds
Fast Yellow AB is similar to other azo dyes such as Acid Yellow 9 and Tartrazine. it is unique due to its specific chemical structure, which includes two sulfonic acid groups and an amino group. This structure gives it distinct properties, such as higher water solubility and specific binding characteristics .
Similar compounds include:
Acid Yellow 9: Another azo dye with similar applications but different chemical structure.
Tartrazine: A widely used food dye with a different chemical structure and regulatory status.
This compound’s unique structure and properties make it a valuable compound for various scientific and industrial applications, despite its restrictions in food use.
Properties
CAS No. |
2706-28-7 |
---|---|
Molecular Formula |
C12H11N3NaO6S2 |
Molecular Weight |
380.4 g/mol |
IUPAC Name |
disodium;2-amino-5-[(4-sulfonatophenyl)diazenyl]benzenesulfonate |
InChI |
InChI=1S/C12H11N3O6S2.Na/c13-11-6-3-9(7-12(11)23(19,20)21)15-14-8-1-4-10(5-2-8)22(16,17)18;/h1-7H,13H2,(H,16,17,18)(H,19,20,21); |
InChI Key |
MDQQLIJKUMAGKB-UHFFFAOYSA-N |
SMILES |
C1=CC(=CC=C1N=NC2=CC(=C(C=C2)N)S(=O)(=O)[O-])S(=O)(=O)[O-].[Na+].[Na+] |
Canonical SMILES |
C1=CC(=CC=C1N=NC2=CC(=C(C=C2)N)S(=O)(=O)O)S(=O)(=O)O.[Na] |
79873-36-2 2706-28-7 74543-21-8 |
|
Pictograms |
Irritant |
Related CAS |
79873-36-2 74543-21-8 |
Synonyms |
C.I. acid yellow 9 |
Origin of Product |
United States |
Retrosynthesis Analysis
AI-Powered Synthesis Planning: Our tool employs the Template_relevance Pistachio, Template_relevance Bkms_metabolic, Template_relevance Pistachio_ringbreaker, Template_relevance Reaxys, Template_relevance Reaxys_biocatalysis model, leveraging a vast database of chemical reactions to predict feasible synthetic routes.
One-Step Synthesis Focus: Specifically designed for one-step synthesis, it provides concise and direct routes for your target compounds, streamlining the synthesis process.
Accurate Predictions: Utilizing the extensive PISTACHIO, BKMS_METABOLIC, PISTACHIO_RINGBREAKER, REAXYS, REAXYS_BIOCATALYSIS database, our tool offers high-accuracy predictions, reflecting the latest in chemical research and data.
Strategy Settings
Precursor scoring | Relevance Heuristic |
---|---|
Min. plausibility | 0.01 |
Model | Template_relevance |
Template Set | Pistachio/Bkms_metabolic/Pistachio_ringbreaker/Reaxys/Reaxys_biocatalysis |
Top-N result to add to graph | 6 |
Feasible Synthetic Routes
Q1: What methods have been explored for the removal of Fast Yellow AB from wastewater?
A: Several methods have been investigated for the removal of this compound from wastewater, with sonophotocatalysis emerging as a promising technique. [] This method utilizes a combination of ultrasound and a photocatalyst, often a semiconductor material, to degrade the dye. Research has shown that using silver-impregnated zinc oxide (ZnO) as a photocatalyst in this process leads to significant degradation of this compound. []
Q2: How effective is sonophotocatalysis in degrading this compound compared to other methods?
A: Sonophotocatalysis exhibits higher degradation efficiency compared to photocatalysis alone. A study comparing the two methods demonstrated that under optimal conditions, sonophotocatalysis achieved 88.9% degradation of this compound in 60 minutes. [] In contrast, photocatalysis only yielded a 33% degradation rate for the same duration. [] This difference highlights the synergistic effect of ultrasound and photocatalysis, leading to enhanced degradation kinetics.
Q3: Beyond its presence as a pollutant, does this compound have any other notable interactions in a catalytic context?
A: Interestingly, this compound, despite being a pollutant itself, has demonstrated the ability to enhance the catalytic reduction of other contaminants, specifically oxyanions and diatrizoate. [] Research indicates that this compound, along with other azo dyes, can significantly increase the reduction kinetics of various contaminants, including nitrate, nitrite, bromate, and perchlorate, in the presence of palladium-based catalysts. [] This enhancement is attributed to a hydrogen atom shuttling mechanism facilitated by the reduced form of this compound (hydrazo dye). []
Disclaimer and Information on In-Vitro Research Products
Please be aware that all articles and product information presented on BenchChem are intended solely for informational purposes. The products available for purchase on BenchChem are specifically designed for in-vitro studies, which are conducted outside of living organisms. In-vitro studies, derived from the Latin term "in glass," involve experiments performed in controlled laboratory settings using cells or tissues. It is important to note that these products are not categorized as medicines or drugs, and they have not received approval from the FDA for the prevention, treatment, or cure of any medical condition, ailment, or disease. We must emphasize that any form of bodily introduction of these products into humans or animals is strictly prohibited by law. It is essential to adhere to these guidelines to ensure compliance with legal and ethical standards in research and experimentation.