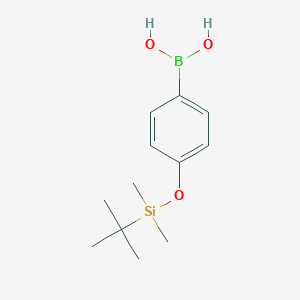
4-(tert-Butyldimethylsilyloxy)phenylboronic acid
Overview
Description
4-(tert-Butyldimethylsilyloxy)phenylboronic acid is an organic compound with the molecular formula C12H21BO3Si and a molecular weight of 252.19 g/mol . It is a boronic acid derivative, characterized by the presence of a phenyl ring substituted with a tert-butyldimethylsilyloxy group and a boronic acid functional group. This compound is commonly used in organic synthesis, particularly in Suzuki-Miyaura coupling reactions .
Mechanism of Action
Target of Action
4-(tert-Butyldimethylsilyloxy)phenylboronic acid is a reactant involved in various chemical reactions . It is used in asymmetric addition reactions with β-substituted cyclic enones, hydroarylation and heterocyclization with phenylpropiolates, and double Suzuki-Miyaura coupling reactions . The primary targets of this compound are the molecules involved in these reactions.
Mode of Action
The compound interacts with its targets through chemical reactions. For instance, in Suzuki-Miyaura coupling reactions, it acts as a boronic acid derivative, which is a key component in these reactions . The resulting changes depend on the specific reaction in which it is involved.
Biochemical Pathways
The compound affects the biochemical pathways associated with the reactions it is involved in. For example, in the synthesis of biologically active molecules such as phenylpyridone derivatives, atromentin and its O-alkylated derivatives, and gelatinases and MT1-MMP inhibitors , it plays a crucial role in the formation of these molecules.
Result of Action
The molecular and cellular effects of the compound’s action depend on the specific reactions it is involved in. For instance, it is used as a starting material for the synthesis of red electroluminescent polyfluorenes . In the synthesis of biologically active molecules, it contributes to the formation of these molecules .
Action Environment
The action, efficacy, and stability of this compound are influenced by environmental factors such as temperature, pH, and the presence of other reactants. For instance, its reactivity in Suzuki-Miyaura coupling reactions would be affected by the presence of a palladium catalyst and a base .
Biochemical Analysis
Biochemical Properties
4-(tert-Butyldimethylsilyloxy)phenylboronic acid plays a significant role in biochemical reactions due to its ability to form reversible covalent bonds with diols and other nucleophiles. This property allows it to interact with various enzymes, proteins, and other biomolecules. For instance, it can inhibit the activity of proteases by binding to the active site serine residue, forming a stable boronate ester. Additionally, this compound can interact with carbohydrate-binding proteins, such as lectins, by forming complexes with the carbohydrate moieties on these proteins .
Cellular Effects
The effects of this compound on cells and cellular processes are diverse. It has been shown to influence cell signaling pathways by modulating the activity of kinases and phosphatases. This compound can also affect gene expression by interacting with transcription factors and other regulatory proteins. Furthermore, this compound can alter cellular metabolism by inhibiting key metabolic enzymes, leading to changes in the levels of various metabolites .
Molecular Mechanism
At the molecular level, this compound exerts its effects through several mechanisms. It can form covalent bonds with the active sites of enzymes, leading to enzyme inhibition or activation. For example, it can inhibit serine proteases by forming a boronate ester with the active site serine residue. Additionally, this compound can bind to transcription factors, altering their ability to regulate gene expression. These interactions can result in changes in cellular function and metabolism .
Temporal Effects in Laboratory Settings
In laboratory settings, the effects of this compound can change over time due to its stability and degradation. This compound is relatively stable under standard laboratory conditions, but it can degrade over time, especially in the presence of moisture or high temperatures. Long-term studies have shown that this compound can have lasting effects on cellular function, including sustained inhibition of enzyme activity and prolonged changes in gene expression .
Dosage Effects in Animal Models
The effects of this compound in animal models vary with different dosages. At low doses, it can modulate enzyme activity and cellular signaling pathways without causing significant toxicity. At higher doses, it can lead to adverse effects, including toxicity and disruption of normal cellular functions. Studies have shown that there is a threshold dose above which the compound’s toxic effects become pronounced, leading to cell death and tissue damage .
Metabolic Pathways
This compound is involved in several metabolic pathways. It can interact with enzymes involved in carbohydrate metabolism, such as glycosidases and glycosyltransferases, by forming reversible covalent bonds with their active sites. This interaction can affect the metabolic flux and levels of various metabolites. Additionally, this compound can be metabolized by liver enzymes, leading to the formation of various metabolites that can be further studied for their biological activity .
Transport and Distribution
Within cells and tissues, this compound is transported and distributed through interactions with transporters and binding proteins. It can be taken up by cells via specific transporters and can bind to intracellular proteins, affecting its localization and accumulation. The compound’s distribution within tissues can also be influenced by its ability to form complexes with extracellular matrix components .
Subcellular Localization
The subcellular localization of this compound is determined by its interactions with various cellular components. It can be directed to specific compartments or organelles through targeting signals or post-translational modifications. For example, it can be localized to the nucleus by binding to nuclear transport proteins or to the endoplasmic reticulum by interacting with ER-resident proteins. These localizations can affect the compound’s activity and function within the cell .
Preparation Methods
4-(tert-Butyldimethylsilyloxy)phenylboronic acid can be synthesized through the reaction of 4-hydroxyphenylboronic acid with tert-butyldimethylchlorosilane under basic conditions . The reaction typically involves the use of a base such as triethylamine to facilitate the formation of the silyl ether. The reaction is carried out in an organic solvent like dichloromethane at room temperature, followed by purification through recrystallization .
Chemical Reactions Analysis
4-(tert-Butyldimethylsilyloxy)phenylboronic acid undergoes various types of chemical reactions, including:
Suzuki-Miyaura Coupling Reactions: This compound is commonly used in Suzuki-Miyaura coupling reactions, where it reacts with aryl halides in the presence of a palladium catalyst and a base to form biaryl compounds.
Hydroarylation and Heterocyclization: It participates in hydroarylation and heterocyclization reactions with phenylpropiolates.
Asymmetric Addition Reactions: It is involved in asymmetric addition reactions with β-substituted cyclic enones.
Common reagents used in these reactions include palladium catalysts, bases such as potassium carbonate, and solvents like toluene or ethanol. The major products formed from these reactions are typically biaryl compounds, which are valuable intermediates in the synthesis of pharmaceuticals and organic materials .
Scientific Research Applications
4-(tert-Butyldimethylsilyloxy)phenylboronic acid has a wide range of applications in scientific research:
Organic Synthesis: It is used as a reactant in the synthesis of red electroluminescent polyfluorenes, which are materials used in organic light-emitting diodes (OLEDs).
Biologically Active Molecules: It serves as a starting material for the synthesis of biologically active molecules, including phenylpyridone derivatives as MCH1R antagonists and gelatinase inhibitors.
Comparison with Similar Compounds
4-(tert-Butyldimethylsilyloxy)phenylboronic acid can be compared with other boronic acid derivatives, such as:
Phenylboronic Acid: Lacks the silyloxy protecting group, making it less stable in certain reactions.
4-Methoxyphenylboronic Acid: Contains a methoxy group instead of a silyloxy group, which can influence its reactivity and selectivity in organic reactions.
4-tert-Butylphenylboronic Acid: Similar structure but without the silyloxy group, affecting its solubility and reactivity.
The presence of the tert-butyldimethylsilyloxy group in this compound provides unique stability and reactivity, making it a valuable compound in organic synthesis .
Properties
IUPAC Name |
[4-[tert-butyl(dimethyl)silyl]oxyphenyl]boronic acid | |
---|---|---|
Source | PubChem | |
URL | https://pubchem.ncbi.nlm.nih.gov | |
Description | Data deposited in or computed by PubChem | |
InChI |
InChI=1S/C12H21BO3Si/c1-12(2,3)17(4,5)16-11-8-6-10(7-9-11)13(14)15/h6-9,14-15H,1-5H3 | |
Source | PubChem | |
URL | https://pubchem.ncbi.nlm.nih.gov | |
Description | Data deposited in or computed by PubChem | |
InChI Key |
NVHHEADQQACSCJ-UHFFFAOYSA-N | |
Source | PubChem | |
URL | https://pubchem.ncbi.nlm.nih.gov | |
Description | Data deposited in or computed by PubChem | |
Canonical SMILES |
B(C1=CC=C(C=C1)O[Si](C)(C)C(C)(C)C)(O)O | |
Source | PubChem | |
URL | https://pubchem.ncbi.nlm.nih.gov | |
Description | Data deposited in or computed by PubChem | |
Molecular Formula |
C12H21BO3Si | |
Source | PubChem | |
URL | https://pubchem.ncbi.nlm.nih.gov | |
Description | Data deposited in or computed by PubChem | |
DSSTOX Substance ID |
DTXSID90455505 | |
Record name | 4-(tert-Butyldimethylsilyloxy)phenylboronic acid | |
Source | EPA DSSTox | |
URL | https://comptox.epa.gov/dashboard/DTXSID90455505 | |
Description | DSSTox provides a high quality public chemistry resource for supporting improved predictive toxicology. | |
Molecular Weight |
252.19 g/mol | |
Source | PubChem | |
URL | https://pubchem.ncbi.nlm.nih.gov | |
Description | Data deposited in or computed by PubChem | |
CAS No. |
159191-56-7 | |
Record name | 4-(tert-Butyldimethylsilyloxy)phenylboronic acid | |
Source | EPA DSSTox | |
URL | https://comptox.epa.gov/dashboard/DTXSID90455505 | |
Description | DSSTox provides a high quality public chemistry resource for supporting improved predictive toxicology. | |
Record name | 4-(tert-Butyldimethylsilyloxy)phenylboronic Acid (contains varying amounts of Anhydride) | |
Source | European Chemicals Agency (ECHA) | |
URL | https://echa.europa.eu/information-on-chemicals | |
Description | The European Chemicals Agency (ECHA) is an agency of the European Union which is the driving force among regulatory authorities in implementing the EU's groundbreaking chemicals legislation for the benefit of human health and the environment as well as for innovation and competitiveness. | |
Explanation | Use of the information, documents and data from the ECHA website is subject to the terms and conditions of this Legal Notice, and subject to other binding limitations provided for under applicable law, the information, documents and data made available on the ECHA website may be reproduced, distributed and/or used, totally or in part, for non-commercial purposes provided that ECHA is acknowledged as the source: "Source: European Chemicals Agency, http://echa.europa.eu/". Such acknowledgement must be included in each copy of the material. ECHA permits and encourages organisations and individuals to create links to the ECHA website under the following cumulative conditions: Links can only be made to webpages that provide a link to the Legal Notice page. | |
Synthesis routes and methods I
Procedure details
Synthesis routes and methods II
Procedure details
Synthesis routes and methods III
Procedure details
Retrosynthesis Analysis
AI-Powered Synthesis Planning: Our tool employs the Template_relevance Pistachio, Template_relevance Bkms_metabolic, Template_relevance Pistachio_ringbreaker, Template_relevance Reaxys, Template_relevance Reaxys_biocatalysis model, leveraging a vast database of chemical reactions to predict feasible synthetic routes.
One-Step Synthesis Focus: Specifically designed for one-step synthesis, it provides concise and direct routes for your target compounds, streamlining the synthesis process.
Accurate Predictions: Utilizing the extensive PISTACHIO, BKMS_METABOLIC, PISTACHIO_RINGBREAKER, REAXYS, REAXYS_BIOCATALYSIS database, our tool offers high-accuracy predictions, reflecting the latest in chemical research and data.
Strategy Settings
Precursor scoring | Relevance Heuristic |
---|---|
Min. plausibility | 0.01 |
Model | Template_relevance |
Template Set | Pistachio/Bkms_metabolic/Pistachio_ringbreaker/Reaxys/Reaxys_biocatalysis |
Top-N result to add to graph | 6 |
Feasible Synthetic Routes
Disclaimer and Information on In-Vitro Research Products
Please be aware that all articles and product information presented on BenchChem are intended solely for informational purposes. The products available for purchase on BenchChem are specifically designed for in-vitro studies, which are conducted outside of living organisms. In-vitro studies, derived from the Latin term "in glass," involve experiments performed in controlled laboratory settings using cells or tissues. It is important to note that these products are not categorized as medicines or drugs, and they have not received approval from the FDA for the prevention, treatment, or cure of any medical condition, ailment, or disease. We must emphasize that any form of bodily introduction of these products into humans or animals is strictly prohibited by law. It is essential to adhere to these guidelines to ensure compliance with legal and ethical standards in research and experimentation.