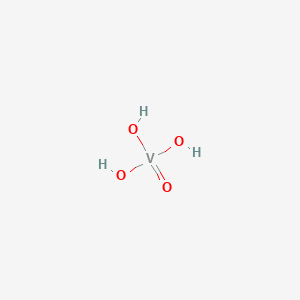
Vanadic acid
Overview
Description
Orthovanadate is an inorganic compound with the chemical formula Na₃VO₄. It is a salt of the VO₄³⁻ oxyanion and is typically found as a colorless, water-soluble solid. Orthovanadate compounds are known for their structural similarity to phosphates, which allows them to interact with various biological systems .
Preparation Methods
Orthovanadate can be synthesized through several methods. One common synthetic route involves dissolving vanadium (V) oxide in a solution of sodium hydroxide. The reaction is as follows:
V2O5+6NaOH→2Na3VO4+3H2O
In industrial settings, orthovanadate is often produced by similar methods, ensuring high purity and yield. The compound can also be prepared by adjusting the pH of a sodium orthovanadate solution to around 10 and boiling it to depolymerize decavanadate into the active monovanadate form .
Chemical Reactions Analysis
Orthovanadate undergoes various chemical reactions, including oxidation, reduction, and substitution reactions. At high pH levels, VO₄³⁻ ions exist in equilibrium with HVO₄²⁻. At lower pH levels, condensation reactions occur, leading to the formation of polyoxovanadates, and ultimately, decavanadate .
Common reagents used in these reactions include sodium hydroxide for synthesis and ethylenediaminetetraacetic acid (EDTA) for reversing inhibitory effects. Major products formed from these reactions include different polyoxovanadates and monovanadate .
Scientific Research Applications
Orthovanadate has a wide range of scientific research applications:
Mechanism of Action
Orthovanadate exerts its effects by mimicking phosphate ions, allowing it to competitively inhibit enzymes such as ATPases and phosphatases. This inhibition can be reversed by dilution or the addition of ethylenediaminetetraacetic acid (EDTA). The compound is activated by boiling and adjusting the pH to around 10, which depolymerizes decavanadate into the active monovanadate form .
Comparison with Similar Compounds
Orthovanadate is often compared with other vanadate compounds, such as metavanadate and decavanadate. While all these compounds contain vanadium in different oxidation states and coordination environments, orthovanadate is unique due to its structural similarity to phosphates, making it particularly effective as an enzyme inhibitor .
Similar compounds include:
Metavanadate (VO₃⁻): Typically found in acidic solutions and forms different polyoxovanadates upon condensation.
Decavanadate (V₁₀O₂₈⁶⁻): Formed at lower pH levels and known for its complex structure and biological activity.
Orthovanadate’s unique properties and versatility make it a valuable compound in various scientific and industrial applications.
Properties
Molecular Formula |
H3O4V |
---|---|
Molecular Weight |
117.963 g/mol |
IUPAC Name |
trihydroxy(oxo)vanadium |
InChI |
InChI=1S/3H2O.O.V/h3*1H2;;/q;;;;+3/p-3 |
InChI Key |
WQEVDHBJGNOKKO-UHFFFAOYSA-K |
SMILES |
O[V](=O)(O)O |
Canonical SMILES |
O[V](=O)(O)O |
Origin of Product |
United States |
Synthesis routes and methods
Procedure details
Disclaimer and Information on In-Vitro Research Products
Please be aware that all articles and product information presented on BenchChem are intended solely for informational purposes. The products available for purchase on BenchChem are specifically designed for in-vitro studies, which are conducted outside of living organisms. In-vitro studies, derived from the Latin term "in glass," involve experiments performed in controlled laboratory settings using cells or tissues. It is important to note that these products are not categorized as medicines or drugs, and they have not received approval from the FDA for the prevention, treatment, or cure of any medical condition, ailment, or disease. We must emphasize that any form of bodily introduction of these products into humans or animals is strictly prohibited by law. It is essential to adhere to these guidelines to ensure compliance with legal and ethical standards in research and experimentation.