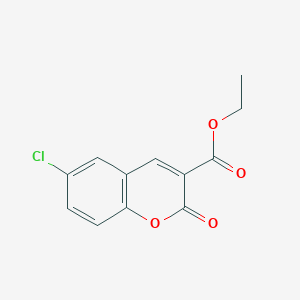
Ethyl 6-chloro-2-oxo-2H-chromene-3-carboxylate
Overview
Description
Ethyl 6-chloro-2-oxo-2H-chromene-3-carboxylate is a chemical compound belonging to the class of chromene derivatives Chromenes are known for their diverse biological activities and are widely used in medicinal chemistry
Preparation Methods
Synthetic Routes and Reaction Conditions
The synthesis of Ethyl 6-chloro-2-oxo-2H-chromene-3-carboxylate typically involves the reaction of 6-chlorocoumarin with ethyl chloroformate in the presence of a base such as sodium hydride or potassium carbonate. The reaction is carried out in an organic solvent like dichloromethane or tetrahydrofuran at room temperature or slightly elevated temperatures. The product is then purified using standard techniques such as recrystallization or column chromatography .
Industrial Production Methods
On an industrial scale, the production of this compound follows similar synthetic routes but with optimized reaction conditions to ensure higher yields and purity. Continuous flow reactors and automated systems are often employed to enhance efficiency and scalability .
Chemical Reactions Analysis
Types of Reactions
Ethyl 6-chloro-2-oxo-2H-chromene-3-carboxylate undergoes various chemical reactions, including:
Substitution Reactions: The chlorine atom can be substituted with other nucleophiles such as amines or thiols.
Reduction Reactions: The carbonyl group can be reduced to a hydroxyl group using reducing agents like sodium borohydride.
Oxidation Reactions: The compound can be oxidized to form more complex derivatives.
Common Reagents and Conditions
Substitution: Nucleophiles like amines or thiols in the presence of a base such as triethylamine.
Reduction: Sodium borohydride in methanol or ethanol.
Oxidation: Oxidizing agents like potassium permanganate or chromium trioxide.
Major Products
Substitution: Formation of amino or thio derivatives.
Reduction: Formation of the corresponding alcohol.
Oxidation: Formation of more oxidized chromene derivatives.
Scientific Research Applications
Ethyl 6-chloro-2-oxo-2H-chromene-3-carboxylate has several applications in scientific research:
Chemistry: Used as an intermediate in the synthesis of more complex organic molecules.
Biology: Studied for its potential biological activities, including antimicrobial and anticancer properties.
Medicine: Investigated for its potential use in drug development, particularly in the design of new therapeutic agents.
Industry: Utilized in the production of dyes, pigments, and other industrial chemicals.
Mechanism of Action
The mechanism of action of Ethyl 6-chloro-2-oxo-2H-chromene-3-carboxylate involves its interaction with various molecular targets. The compound can inhibit specific enzymes or receptors, leading to its biological effects. For example, it may inhibit certain kinases involved in cell signaling pathways, thereby exerting its anticancer properties .
Comparison with Similar Compounds
Similar Compounds
- Ethyl 6-bromo-2-oxo-2H-chromene-3-carboxylate
- Ethyl 6-fluoro-2-oxo-2H-chromene-3-carboxylate
- Ethyl 6-methyl-2-oxo-2H-chromene-3-carboxylate
Uniqueness
Ethyl 6-chloro-2-oxo-2H-chromene-3-carboxylate is unique due to the presence of the chlorine atom, which can significantly influence its reactivity and biological activity. The chlorine atom can participate in various substitution reactions, making it a versatile intermediate in organic synthesis .
Properties
IUPAC Name |
ethyl 6-chloro-2-oxochromene-3-carboxylate | |
---|---|---|
Source | PubChem | |
URL | https://pubchem.ncbi.nlm.nih.gov | |
Description | Data deposited in or computed by PubChem | |
InChI |
InChI=1S/C12H9ClO4/c1-2-16-11(14)9-6-7-5-8(13)3-4-10(7)17-12(9)15/h3-6H,2H2,1H3 | |
Source | PubChem | |
URL | https://pubchem.ncbi.nlm.nih.gov | |
Description | Data deposited in or computed by PubChem | |
InChI Key |
HDBCARVARPQBKI-UHFFFAOYSA-N | |
Source | PubChem | |
URL | https://pubchem.ncbi.nlm.nih.gov | |
Description | Data deposited in or computed by PubChem | |
Canonical SMILES |
CCOC(=O)C1=CC2=C(C=CC(=C2)Cl)OC1=O | |
Source | PubChem | |
URL | https://pubchem.ncbi.nlm.nih.gov | |
Description | Data deposited in or computed by PubChem | |
Molecular Formula |
C12H9ClO4 | |
Source | PubChem | |
URL | https://pubchem.ncbi.nlm.nih.gov | |
Description | Data deposited in or computed by PubChem | |
DSSTOX Substance ID |
DTXSID60345146 | |
Record name | Ethyl 6-chloro-2-oxo-2H-chromene-3-carboxylate | |
Source | EPA DSSTox | |
URL | https://comptox.epa.gov/dashboard/DTXSID60345146 | |
Description | DSSTox provides a high quality public chemistry resource for supporting improved predictive toxicology. | |
Molecular Weight |
252.65 g/mol | |
Source | PubChem | |
URL | https://pubchem.ncbi.nlm.nih.gov | |
Description | Data deposited in or computed by PubChem | |
CAS No. |
70384-80-4 | |
Record name | Ethyl 6-chloro-2-oxo-2H-chromene-3-carboxylate | |
Source | EPA DSSTox | |
URL | https://comptox.epa.gov/dashboard/DTXSID60345146 | |
Description | DSSTox provides a high quality public chemistry resource for supporting improved predictive toxicology. | |
Retrosynthesis Analysis
AI-Powered Synthesis Planning: Our tool employs the Template_relevance Pistachio, Template_relevance Bkms_metabolic, Template_relevance Pistachio_ringbreaker, Template_relevance Reaxys, Template_relevance Reaxys_biocatalysis model, leveraging a vast database of chemical reactions to predict feasible synthetic routes.
One-Step Synthesis Focus: Specifically designed for one-step synthesis, it provides concise and direct routes for your target compounds, streamlining the synthesis process.
Accurate Predictions: Utilizing the extensive PISTACHIO, BKMS_METABOLIC, PISTACHIO_RINGBREAKER, REAXYS, REAXYS_BIOCATALYSIS database, our tool offers high-accuracy predictions, reflecting the latest in chemical research and data.
Strategy Settings
Precursor scoring | Relevance Heuristic |
---|---|
Min. plausibility | 0.01 |
Model | Template_relevance |
Template Set | Pistachio/Bkms_metabolic/Pistachio_ringbreaker/Reaxys/Reaxys_biocatalysis |
Top-N result to add to graph | 6 |
Feasible Synthetic Routes
Disclaimer and Information on In-Vitro Research Products
Please be aware that all articles and product information presented on BenchChem are intended solely for informational purposes. The products available for purchase on BenchChem are specifically designed for in-vitro studies, which are conducted outside of living organisms. In-vitro studies, derived from the Latin term "in glass," involve experiments performed in controlled laboratory settings using cells or tissues. It is important to note that these products are not categorized as medicines or drugs, and they have not received approval from the FDA for the prevention, treatment, or cure of any medical condition, ailment, or disease. We must emphasize that any form of bodily introduction of these products into humans or animals is strictly prohibited by law. It is essential to adhere to these guidelines to ensure compliance with legal and ethical standards in research and experimentation.