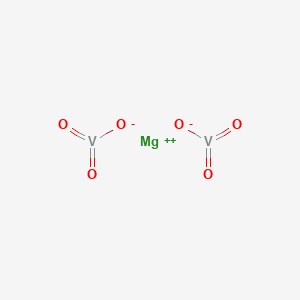
Magnesium;oxido(dioxo)vanadium
- Click on QUICK INQUIRY to receive a quote from our team of experts.
- With the quality product at a COMPETITIVE price, you can focus more on your research.
Overview
Description
Magnesium;oxido(dioxo)vanadium is a compound that consists of magnesium, vanadium, and oxygenThe compound is part of the broader family of vanadium oxides, which are notable for their diverse oxidation states and structural versatility .
Preparation Methods
Synthetic Routes and Reaction Conditions: Magnesium;oxido(dioxo)vanadium can be synthesized through various methods. One common approach involves the solid-state reaction between magnesium oxide and vanadium pentoxide. The reaction typically occurs at high temperatures to ensure complete conversion of the reactants into the desired product .
Industrial Production Methods: In industrial settings, the preparation of this compound often involves the use of hydrothermal methods. This approach allows for better control over the particle size and morphology of the final product. The reaction conditions, such as temperature, pressure, and reaction time, are carefully optimized to achieve high purity and yield .
Chemical Reactions Analysis
Types of Reactions: Magnesium;oxido(dioxo)vanadium undergoes various chemical reactions, including oxidation, reduction, and substitution reactions. These reactions are influenced by the oxidation states of vanadium and the presence of other reactants.
Common Reagents and Conditions:
Oxidation: The compound can be oxidized using strong oxidizing agents such as hydrogen peroxide or potassium permanganate.
Reduction: Reduction reactions can be carried out using reducing agents like sodium borohydride or hydrogen gas.
Substitution: Substitution reactions often involve the replacement of oxygen atoms with other ligands, such as halides or organic groups.
Major Products Formed: The major products formed from these reactions depend on the specific conditions and reagents used. For example, oxidation reactions may yield higher oxidation state vanadium compounds, while reduction reactions can produce lower oxidation state vanadium species .
Scientific Research Applications
Magnesium;oxido(dioxo)vanadium has several scientific research applications:
Mechanism of Action
The mechanism of action of magnesium;oxido(dioxo)vanadium involves its ability to undergo redox reactions, which are crucial for its applications in catalysis and energy storage. The compound can interact with various molecular targets, including enzymes and cellular components, through its redox-active vanadium centers. These interactions can lead to the activation or inhibition of specific biochemical pathways .
Comparison with Similar Compounds
Vanadium Pentoxide (V2O5): Known for its high catalytic activity in oxidation reactions.
Vanadium Dioxide (VO2): Notable for its reversible metal-to-insulator phase transition.
Magnesium Vanadate (Mg3V2O8): Studied for its luminescent properties and potential use in energy storage.
Uniqueness: Magnesium;oxido(dioxo)vanadium stands out due to its combination of magnesium and vanadium, which imparts unique electrochemical properties. This makes it particularly suitable for applications in rechargeable batteries and catalysis, where both high redox activity and structural stability are required .
Properties
Molecular Formula |
MgO6V2 |
---|---|
Molecular Weight |
222.18 g/mol |
IUPAC Name |
magnesium;oxido(dioxo)vanadium |
InChI |
InChI=1S/Mg.6O.2V/q+2;;;;;2*-1;; |
InChI Key |
UQTWTHDGOFVKGC-UHFFFAOYSA-N |
Canonical SMILES |
[O-][V](=O)=O.[O-][V](=O)=O.[Mg+2] |
Origin of Product |
United States |
Disclaimer and Information on In-Vitro Research Products
Please be aware that all articles and product information presented on BenchChem are intended solely for informational purposes. The products available for purchase on BenchChem are specifically designed for in-vitro studies, which are conducted outside of living organisms. In-vitro studies, derived from the Latin term "in glass," involve experiments performed in controlled laboratory settings using cells or tissues. It is important to note that these products are not categorized as medicines or drugs, and they have not received approval from the FDA for the prevention, treatment, or cure of any medical condition, ailment, or disease. We must emphasize that any form of bodily introduction of these products into humans or animals is strictly prohibited by law. It is essential to adhere to these guidelines to ensure compliance with legal and ethical standards in research and experimentation.