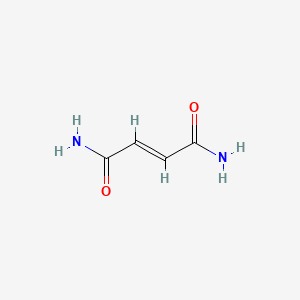
Fumaramide
Overview
Description
Fumaramide, also known as trans-butenediamide, is an organic compound with the chemical formula C4H6N2O2. It appears as a white crystalline powder and is primarily used as an intermediate in organic synthesis. This compound is derived from fumaric acid and is known for its role in various chemical reactions and industrial applications.
Mechanism of Action
Target of Action
Fumaramide primarily targets the N-benzyl group of the thread by the amidate group of the macrocycle . This interaction plays a crucial role in the cyclization process of interlocked fumaramides .
Mode of Action
The mode of action of this compound involves a diastereoselective CsOH-promoted cyclization of interlocked fumaramides to give β-lactams . The rate-determining step in this process is the deprotonation of the N-benzyl group of the thread by the amidate group of the macrocycle generated by the external base . This interaction results in significant changes in the structure and reactivity of the compound .
Biochemical Pathways
The biochemical pathways affected by this compound involve the cyclization of interlocked fumaramides into β-lactams . The higher flexibility of the adamantyl core speeds up the cyclization process in diadamantyl-derived rotaxanes .
Result of Action
The molecular and cellular effects of this compound’s action involve the formation of β-lactams through a diastereoselective cyclization process . This process is influenced by the deprotonation of the N-benzyl group of the thread by the amidate group of the macrocycle .
Action Environment
The action of this compound is influenced by environmental factors such as the presence of an external base . The interlocked polyamide macrocycle plays a definitive activating role, making it an exception to the rule of interlocked systems in which the macrocycle effect alters the reaction outcomes .
Biochemical Analysis
Biochemical Properties
Fumaramide plays a significant role in various biochemical reactions. It interacts with several enzymes, proteins, and other biomolecules. For instance, this compound derivatives have been evaluated as inhibitors of acetylcholinesterase (AChE) and butyrylcholinesterase (BuChE), enzymes linked to Alzheimer’s disease . These interactions are crucial as they can modulate the activity of these enzymes, potentially leading to therapeutic applications.
Cellular Effects
This compound influences various cellular processes and functions. It has been shown to affect cell signaling pathways, gene expression, and cellular metabolism. For example, this compound derivatives have been studied for their potential to inhibit acetylcholinesterase, which plays a role in neurotransmission . This inhibition can impact cell signaling and neurotransmitter levels, thereby influencing cellular functions.
Molecular Mechanism
The molecular mechanism of this compound involves its interaction with specific biomolecules. This compound compounds have been found to target both the catalytic anionic site and the peripheral anionic site of acetylcholinesterase . This dual targeting mechanism allows this compound to effectively inhibit the enzyme, thereby modulating its activity. Additionally, the α,β-unsaturated 1,4-diketone moiety in this compound compounds is crucial for their inhibitory activity .
Temporal Effects in Laboratory Settings
In laboratory settings, the effects of this compound can change over time. Studies have shown that the stability and degradation of this compound can influence its long-term effects on cellular function. For instance, the cyclization of interlocked fumaramides into β-lactams has been studied to understand the temporal changes in their activity . These studies help in understanding the stability and degradation patterns of this compound over time.
Dosage Effects in Animal Models
The effects of this compound vary with different dosages in animal models. Higher doses of this compound can lead to toxic or adverse effects, while lower doses may be therapeutic. For example, studies on the dosage effects of various compounds, including this compound, have shown that the pharmacokinetics and metabolism can differ significantly between species . Understanding these dosage effects is crucial for determining the therapeutic window and potential toxicity of this compound.
Metabolic Pathways
This compound is involved in several metabolic pathways. It interacts with enzymes and cofactors that modulate metabolic flux and metabolite levels. For instance, the inhibition of fumarate hydratase by this compound can lead to increased fumarate levels and suppression of mitochondrial respiration . This interaction highlights the role of this compound in metabolic regulation and its potential impact on cellular metabolism.
Transport and Distribution
The transport and distribution of this compound within cells and tissues are mediated by specific transporters and binding proteins. Studies have shown that this compound can be transported across cell membranes and distributed to various cellular compartments . Understanding the transport mechanisms of this compound is essential for determining its bioavailability and therapeutic potential.
Subcellular Localization
This compound is localized in specific subcellular compartments, which can influence its activity and function. For example, fumarase, a related enzyme, is primarily localized in the mitochondria but can also be found in other cellular compartments . The subcellular localization of this compound can affect its interactions with other biomolecules and its overall activity within the cell.
Preparation Methods
Synthetic Routes and Reaction Conditions: Fumaramide can be synthesized through the reaction of diethyl fumarate with concentrated ammonium hydroxide. The reaction mixture is stirred at 25–30°C for several hours, resulting in the formation of this compound. The product is then filtered, washed with water, and dried .
Industrial Production Methods: In an industrial setting, this compound is produced by reacting diethyl fumarate with ammonia in the presence of a catalyst. The reaction is carried out under controlled temperature and pressure conditions to ensure high yield and purity of the product. The resulting this compound is then purified through recrystallization or other suitable methods.
Chemical Reactions Analysis
Types of Reactions: Fumaramide undergoes various chemical reactions, including cyclization, substitution, and reduction. One notable reaction is the CsOH-promoted cyclization of interlocked fumaramides to form β-lactams .
Common Reagents and Conditions:
Cyclization: CsOH is used as a base to promote the cyclization of fumaramides into β-lactams.
Substitution: this compound can undergo substitution reactions with various nucleophiles, such as amines and alcohols, under mild conditions.
Reduction: this compound can be reduced to form the corresponding amine using reducing agents like lithium aluminum hydride (LiAlH4) or sodium borohydride (NaBH4).
Major Products:
β-Lactams: Formed through the cyclization of fumaramides.
Substituted Amides: Formed through substitution reactions with nucleophiles.
Amines: Formed through the reduction of this compound.
Scientific Research Applications
Fumaramide has several applications in scientific research, including:
Medicine: this compound derivatives have shown promise as potential therapeutic agents due to their biological activity.
Industry: this compound is used as an intermediate in the production of pharmaceuticals, agrochemicals, and other fine chemicals.
Comparison with Similar Compounds
Oxamide: Similar to fumaramide, oxamide is an amide derivative with the chemical formula C2H4N2O2.
Fumaric Acid: The parent compound of this compound, fumaric acid, is a dicarboxylic acid with the chemical formula C4H4O4. This compound is derived from fumaric acid through the reaction with ammonia.
Uniqueness of this compound: this compound’s unique properties, such as its ability to undergo cyclization to form β-lactams and its effectiveness as an enzyme inhibitor, distinguish it from other similar compounds. The presence of an ethylene bridge in this compound derivatives enhances their biological activity compared to oxamide derivatives .
Properties
IUPAC Name |
(E)-but-2-enediamide | |
---|---|---|
Source | PubChem | |
URL | https://pubchem.ncbi.nlm.nih.gov | |
Description | Data deposited in or computed by PubChem | |
InChI |
InChI=1S/C4H6N2O2/c5-3(7)1-2-4(6)8/h1-2H,(H2,5,7)(H2,6,8)/b2-1+ | |
Source | PubChem | |
URL | https://pubchem.ncbi.nlm.nih.gov | |
Description | Data deposited in or computed by PubChem | |
InChI Key |
BSSNZUFKXJJCBG-OWOJBTEDSA-N | |
Source | PubChem | |
URL | https://pubchem.ncbi.nlm.nih.gov | |
Description | Data deposited in or computed by PubChem | |
Canonical SMILES |
C(=CC(=O)N)C(=O)N | |
Source | PubChem | |
URL | https://pubchem.ncbi.nlm.nih.gov | |
Description | Data deposited in or computed by PubChem | |
Isomeric SMILES |
C(=C/C(=O)N)\C(=O)N | |
Source | PubChem | |
URL | https://pubchem.ncbi.nlm.nih.gov | |
Description | Data deposited in or computed by PubChem | |
Molecular Formula |
C4H6N2O2 | |
Source | PubChem | |
URL | https://pubchem.ncbi.nlm.nih.gov | |
Description | Data deposited in or computed by PubChem | |
DSSTOX Substance ID |
DTXSID201318367 | |
Record name | Fumaramide | |
Source | EPA DSSTox | |
URL | https://comptox.epa.gov/dashboard/DTXSID201318367 | |
Description | DSSTox provides a high quality public chemistry resource for supporting improved predictive toxicology. | |
Molecular Weight |
114.10 g/mol | |
Source | PubChem | |
URL | https://pubchem.ncbi.nlm.nih.gov | |
Description | Data deposited in or computed by PubChem | |
CAS No. |
627-64-5 | |
Record name | Fumaramide | |
Source | CAS Common Chemistry | |
URL | https://commonchemistry.cas.org/detail?cas_rn=627-64-5 | |
Description | CAS Common Chemistry is an open community resource for accessing chemical information. Nearly 500,000 chemical substances from CAS REGISTRY cover areas of community interest, including common and frequently regulated chemicals, and those relevant to high school and undergraduate chemistry classes. This chemical information, curated by our expert scientists, is provided in alignment with our mission as a division of the American Chemical Society. | |
Explanation | The data from CAS Common Chemistry is provided under a CC-BY-NC 4.0 license, unless otherwise stated. | |
Record name | Fumaramide | |
Source | ChemIDplus | |
URL | https://pubchem.ncbi.nlm.nih.gov/substance/?source=chemidplus&sourceid=0000627645 | |
Description | ChemIDplus is a free, web search system that provides access to the structure and nomenclature authority files used for the identification of chemical substances cited in National Library of Medicine (NLM) databases, including the TOXNET system. | |
Record name | Fumaramide | |
Source | DTP/NCI | |
URL | https://dtp.cancer.gov/dtpstandard/servlet/dwindex?searchtype=NSC&outputformat=html&searchlist=16608 | |
Description | The NCI Development Therapeutics Program (DTP) provides services and resources to the academic and private-sector research communities worldwide to facilitate the discovery and development of new cancer therapeutic agents. | |
Explanation | Unless otherwise indicated, all text within NCI products is free of copyright and may be reused without our permission. Credit the National Cancer Institute as the source. | |
Record name | Fumaramide | |
Source | EPA DSSTox | |
URL | https://comptox.epa.gov/dashboard/DTXSID201318367 | |
Description | DSSTox provides a high quality public chemistry resource for supporting improved predictive toxicology. | |
Record name | Fumaramide | |
Source | European Chemicals Agency (ECHA) | |
URL | https://echa.europa.eu/substance-information/-/substanceinfo/100.010.007 | |
Description | The European Chemicals Agency (ECHA) is an agency of the European Union which is the driving force among regulatory authorities in implementing the EU's groundbreaking chemicals legislation for the benefit of human health and the environment as well as for innovation and competitiveness. | |
Explanation | Use of the information, documents and data from the ECHA website is subject to the terms and conditions of this Legal Notice, and subject to other binding limitations provided for under applicable law, the information, documents and data made available on the ECHA website may be reproduced, distributed and/or used, totally or in part, for non-commercial purposes provided that ECHA is acknowledged as the source: "Source: European Chemicals Agency, http://echa.europa.eu/". Such acknowledgement must be included in each copy of the material. ECHA permits and encourages organisations and individuals to create links to the ECHA website under the following cumulative conditions: Links can only be made to webpages that provide a link to the Legal Notice page. | |
Retrosynthesis Analysis
AI-Powered Synthesis Planning: Our tool employs the Template_relevance Pistachio, Template_relevance Bkms_metabolic, Template_relevance Pistachio_ringbreaker, Template_relevance Reaxys, Template_relevance Reaxys_biocatalysis model, leveraging a vast database of chemical reactions to predict feasible synthetic routes.
One-Step Synthesis Focus: Specifically designed for one-step synthesis, it provides concise and direct routes for your target compounds, streamlining the synthesis process.
Accurate Predictions: Utilizing the extensive PISTACHIO, BKMS_METABOLIC, PISTACHIO_RINGBREAKER, REAXYS, REAXYS_BIOCATALYSIS database, our tool offers high-accuracy predictions, reflecting the latest in chemical research and data.
Strategy Settings
Precursor scoring | Relevance Heuristic |
---|---|
Min. plausibility | 0.01 |
Model | Template_relevance |
Template Set | Pistachio/Bkms_metabolic/Pistachio_ringbreaker/Reaxys/Reaxys_biocatalysis |
Top-N result to add to graph | 6 |
Feasible Synthetic Routes
Q1: What is the molecular formula and weight of fumaramide?
A1: this compound, also known as (E)-But-2-enediamide, has the molecular formula C4H6N2O2 and a molecular weight of 114.10 g/mol. []
Q2: What spectroscopic techniques are commonly used to characterize this compound?
A2: Researchers employ various spectroscopic techniques to characterize this compound and its derivatives. These include:
- Nuclear Magnetic Resonance (NMR) Spectroscopy: Provides information about the structure, dynamics, and interactions of this compound-containing molecules, such as rotaxanes. This technique is extensively used to study macrocycle shuttling rates, hydrogen bonding interactions, and conformational changes. [, , , , ]
- Fourier Transform Infrared (FTIR) Spectroscopy: Offers insights into the functional groups present in this compound derivatives and the nature of intermolecular interactions, such as hydrogen bonding. This technique helps characterize the self-assembly processes in supramolecular systems. [, ]
- High-Resolution Electron Energy Loss Spectroscopy (HREELS): Used to study the adsorption behavior of this compound-based rotaxanes and their components on solid surfaces like gold (Au(111)). This technique helps determine molecular orientation and the presence of chemisorption. []
- UV-Vis Spectroscopy: Useful for monitoring photoisomerization processes in this compound-containing molecules, particularly the conversion of this compound to maleamide. [, , , , ]
Q3: How is this compound employed in the construction of rotaxanes?
A3: this compound serves as a versatile building block for constructing mechanically interlocked molecules like rotaxanes. [, , , , , , , ] Its structure, containing a rigid trans-alkene linker and two amide groups capable of hydrogen bonding, facilitates the formation of stable complexes with macrocyclic molecules. This complexation is often exploited in templated syntheses, where the this compound unit acts as a recognition site for macrocycle threading.
Q4: How does the photoisomerization of this compound influence rotaxane behavior?
A4: The photoisomerization of this compound to its cis isomer, maleamide, significantly impacts the properties and behavior of rotaxanes. [, , , , , ] This isomerization alters the spatial arrangement of hydrogen bond donor and acceptor sites within the molecule. This can lead to:
- Controlled Shuttling: The change in hydrogen bonding patterns can trigger the movement of the macrocycle between different recognition sites on the rotaxane thread, enabling light-driven molecular shuttles. [, , , , ]
- Modulation of Activity: Photoisomerization can be used to control the activity of catalytic rotaxanes by bringing reactive groups into proximity or separating them. []
Q5: What role does this compound play in the formation of supramolecular gels?
A5: Certain this compound derivatives exhibit gelation properties due to their ability to self-assemble into fibrous networks that trap solvent molecules. [, , ] The strong and directional intermolecular hydrogen bonding between this compound units is crucial for gel formation. These gels find potential applications in drug delivery, tissue engineering, and as responsive materials.
Q6: How does the incorporation of this compound affect the properties of polymers?
A6: this compound is incorporated into polymers to impart specific properties, such as photocleavability and unique electrical characteristics. [, , , ] For instance, polyamides containing coumarin dimer components, upon UV irradiation, undergo asymmetric photocleavage, leading to the formation of this compound units and the release of 2,2'-dihydroxystilbene. This photocleavable property is valuable in applications requiring controlled degradation or release of encapsulated substances.
Q7: How is computational chemistry used to study this compound-containing systems?
A7: Computational methods are indispensable for understanding and predicting the behavior of this compound-containing systems. Researchers utilize:
- Density Functional Theory (DFT) Calculations: To investigate reaction mechanisms, predict molecular geometries, and calculate electronic properties. [, ]
- Molecular Dynamics (MD) Simulations: To study the dynamic behavior of rotaxanes, explore conformational changes, and investigate interactions with surfaces or solvents. [, ]
- Quantum Mechanics/Molecular Mechanics (QM/MM) Methods: To model complex systems where a quantum mechanical treatment of the this compound unit and its immediate environment is combined with a classical description of the surrounding molecules. []
Q8: What are the key insights from structure-activity relationship (SAR) studies on this compound derivatives?
A8: SAR studies on this compound derivatives highlight the importance of specific structural features for their activity and properties:
- Stereochemistry: The trans configuration of the double bond in this compound is crucial for its activity in many systems, including its role in enzyme activation and rotaxane function. [] The cis isomer, maleamide, typically exhibits different properties.
- Substitution Pattern: Modifications to the amide groups or the alkene linker can significantly influence the hydrogen bonding ability, conformational flexibility, and overall activity of this compound derivatives. [, , ]
Q9: Are there any biological applications of this compound derivatives?
A9: this compound derivatives have been investigated for various biological applications, including:
- Antibiotic Activity: Fumaramidmycin, a naturally occurring this compound derivative, exhibits antibiotic properties. []
- Enzyme Inhibition: Some this compound analogues act as inhibitors of dopamine beta-mono-oxygenase, an enzyme involved in neurotransmitter synthesis. []
Q10: How does the stability of this compound-based materials vary under different conditions?
A10: The stability of this compound-based materials depends on several factors, including temperature, solvent, and exposure to light. [, , ] For instance:
- Thermal Stability: Polyfumaramides generally exhibit high thermal stability but may undergo crosslinking at elevated temperatures. []
- Photostability: The this compound unit is susceptible to photoisomerization to maleamide upon UV irradiation. This property can be advantageous for controlled release applications but detrimental to long-term material stability. [, , , , , ]
- Hydrolytic Stability: Fumaramides are generally stable under neutral conditions but can undergo hydrolysis in the presence of strong acids or bases. []
Disclaimer and Information on In-Vitro Research Products
Please be aware that all articles and product information presented on BenchChem are intended solely for informational purposes. The products available for purchase on BenchChem are specifically designed for in-vitro studies, which are conducted outside of living organisms. In-vitro studies, derived from the Latin term "in glass," involve experiments performed in controlled laboratory settings using cells or tissues. It is important to note that these products are not categorized as medicines or drugs, and they have not received approval from the FDA for the prevention, treatment, or cure of any medical condition, ailment, or disease. We must emphasize that any form of bodily introduction of these products into humans or animals is strictly prohibited by law. It is essential to adhere to these guidelines to ensure compliance with legal and ethical standards in research and experimentation.