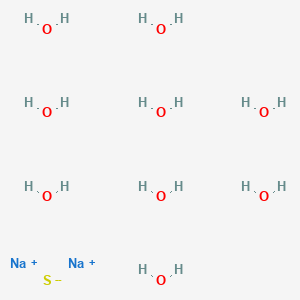
Disodium sulfide nonahydrate
Overview
Description
Disodium sulfide nonahydrate (Na₂S·9H₂O) is a hydrated inorganic compound comprising sodium sulfide coordinated with nine water molecules. It has a molecular formula of H₁₈Na₂O₉S, an average molar mass of 240.175 g/mol, and a monoclinic crystalline structure . This compound is highly soluble in water (15.4 g/100 mL at 10°C, increasing to 57.2 g/mL at 90°C) and exhibits strong alkalinity in aqueous solutions .
Na₂S·9H₂O is widely used in organic synthesis as a sulfurizing agent, reducing agent, and precursor for metal sulfide nanoparticles . It also plays roles in analytical chemistry for ion precipitation , hydrogen sulfide (H₂S) generation in environmental studies , and thermochemical energy storage due to its high enthalpy of hydration . Despite its utility, it is hygroscopic, light-sensitive, and releases toxic H₂S gas upon acidification, necessitating careful handling .
Scientific Research Applications
Scientific Research Applications
-
Source of Hydrogen Sulfide :
Disodium sulfide nonahydrate serves as a reliable source of hydrogen sulfide (H₂S), which is crucial in biochemical research. For instance, it has been employed to study the effects of H₂S on amyloid fibrils related to neurodegenerative diseases, providing insights into potential therapeutic targets for conditions like Alzheimer's disease . -
Analytical Chemistry :
It is used in the preparation of sulfide standards for the quantitative analysis of sulfides in wastewater samples. This application is essential for environmental monitoring and compliance with regulatory standards regarding wastewater management . -
Organic Synthesis :
This compound acts as a catalyst in the synthesis of thioamides and various organic compounds. It facilitates reactions such as the reductive debromination of vic-dibromides to olefins, showcasing its utility in organic chemistry . -
Photochemical Studies :
Research has demonstrated that this compound can produce thiyl radicals via photolysis in phosphate buffers. This property is explored for its potential applications in photochemistry and radical chemistry .
Industrial Applications
-
Textile Industry :
In textile processing, this compound is utilized as a reducing agent during dyeing processes. It enhances dye penetration into fibers, resulting in more vibrant and durable colors . -
Leather Tanning :
The compound plays a significant role in leather manufacturing, particularly in the removal of hair from hides during tanning processes. Its effectiveness in breaking down organic matter ensures high-quality leather production . -
Water Treatment :
This compound is effective in precipitating heavy metals from industrial wastewater, making it an essential component in water purification processes. Its ability to form insoluble metal sulfides aids in meeting environmental discharge standards . -
Mining and Metallurgy :
The compound is used in mineral flotation processes to separate valuable metals such as copper, lead, and zinc from ores. It enhances the efficiency of metal extraction by selectively precipitating impurities . -
Battery Manufacturing :
In the development of sodium-sulfur batteries, this compound contributes to high energy density and efficiency, making it suitable for energy storage applications .
Case Studies
-
Neurodegenerative Disease Research :
A study investigated the influence of hydrogen sulfide on amyloid fibrils using this compound as a H₂S donor. The findings indicated potential pathways for therapeutic intervention in neurodegenerative disorders, emphasizing the compound's relevance in biomedical research . -
Wastewater Treatment Efficacy :
Research conducted on the use of this compound for treating raw sewage demonstrated its capability to effectively reduce sulfide concentrations, thereby improving overall water quality and facilitating compliance with environmental regulations .
Q & A
Basic Research Questions
Q. What are the critical considerations for handling disodium sulfide nonahydrate in laboratory settings?
this compound is hygroscopic, light-sensitive, and releases toxic hydrogen sulfide (H₂S) upon decomposition. Laboratory protocols must include:
- Storage in airtight, light-resistant containers under inert gas (e.g., nitrogen) to minimize oxidation and moisture absorption .
- Use of personal protective equipment (PPE) such as gloves, goggles, and lab coats to prevent skin/eye contact. Waste must be segregated and treated by specialized disposal services due to its reactivity and environmental hazards .
Q. How can researchers verify the purity of this compound for analytical applications?
Purity assessment typically involves iodometric titration (≥98.0% assay) and cation trace analysis (e.g., Fe ≤0.005%, NH₄⁺ ≤0.005%) as per USP/NF and ACS specifications . For advanced applications like LC-MS, additional tests for heavy metals and solubility in glycerol-water mixtures may be required to ensure compatibility with sensitive instrumentation .
Q. What is the standardized method for preparing sulfide ion solutions using this compound?
A stock solution is prepared by dissolving 7.5 g of Na₂S·9H₂O in a 45 mL mixture of water and glycerol (10:29 v/v) under heating, followed by cooling and dilution to 100 mL. This method stabilizes sulfide ions and prevents rapid oxidation, ensuring reproducibility in quantitative analyses .
Advanced Research Questions
Q. How does the concentration of this compound influence the structural and catalytic properties of iron sulfide nanomaterials?
In hydrothermal synthesis (80°C, 6-hour annealing), varying Na₂S·9H₂O concentrations (0.0125–0.1 M) directly modulate sulfide ion availability, which impacts:
- Morphology : Higher concentrations (0.1 M) yield denser iron sulfide precipitates with nano-wall structures, increasing active sites for electrochemical reactions .
- Crystallinity : XRD analysis shows stronger diffraction peaks (e.g., 44.35°, 51.55°) at higher concentrations, correlating with improved crystallinity and sulfur content .
- Electrocatalytic Performance : At 0.1 M, iron sulfide exhibits a low overpotential (260 mV at 10 mA/cm²) and Tafel slope (51 mV/dec) for oxygen evolution reactions (OER), outperforming commercial RuO₂ .
Q. What methodological approaches resolve contradictions in sulfide ion activity during nanomaterial synthesis?
Discrepancies in sulfide reactivity arise from competing hydrolysis (Na₂S + H₂O → 2NaOH + H₂S) and oxidation (2Na₂S + O₂ → 2Na₂SO₃). To mitigate:
- pH Control : Maintain alkaline conditions (pH >10) using NaOH or NH₄OH to suppress H₂S volatilization and stabilize sulfide ions .
- Inert Atmosphere : Conduct reactions under nitrogen/argon to minimize oxidation artifacts .
- Kinetic Monitoring : Use real-time UV-Vis spectroscopy to track sulfide consumption and adjust reagent dosing dynamically .
Q. How do researchers characterize the electrochemical double-layer capacitance (Cdl) of disodium sulfide-derived catalysts?
Cyclic voltammetry (CV) in non-Faradaic regions (e.g., 0.1–0.2 V vs. RHE) is used to calculate Cdl, which correlates with electrochemically active surface area (ECSA). For iron sulfide synthesized with 0.1 M Na₂S·9H₂O, a Cdl of 12.5 mF/cm² indicates a high surface area, enhancing catalytic efficiency for hydrogen evolution reactions (HER) .
Q. Methodological Tables
Table 1: Impact of Na₂S·9H₂O Concentration on Iron Sulfide Properties
Concentration (M) | Color | Sulfur Content (XRD) | Overpotential (OER, mV) | Tafel Slope (mV/dec) |
---|---|---|---|---|
0.0125 | Brownish-yellow | Low | 420 | 89 |
0.025 | Gray | Moderate | 380 | 75 |
0.05 | Blackish-gray | High | 320 | 62 |
0.1 | Blackish-gray | Highest | 260 | 51 |
Data compiled from . |
Table 2: Key Analytical Methods for Na₂S·9H₂O-Derived Materials
Comparison with Similar Compounds
Sodium Sulfide (Anhydrous, Na₂S)
- Chemical Structure: Unlike the nonahydrate, anhydrous Na₂S lacks water molecules, resulting in higher reactivity and instability in humid conditions .
- H₂S Release: Both Na₂S and Na₂S·9H₂O release H₂S rapidly in aqueous media, but the nonahydrate’s water content moderates reaction kinetics, making it preferable in reactions requiring controlled sulfur availability (e.g., synthesis of thieno[2,3-b]pyridines) .
- Applications: Anhydrous Na₂S is less common in wet chemistry due to its sensitivity to moisture, whereas the nonahydrate’s stability in water-containing systems (e.g., DMF with sodium hydride) enhances its utility in organic synthesis .
Sodium Hydrogen Sulfide (NaHS)
- H₂S Release Profile : NaHS releases H₂S faster than Na₂S·9H₂O at physiological pH due to direct dissociation into HS⁻ ions, but both compounds suffer from uncontrollable H₂S burst release, limiting their clinical translatability .
- Solubility : NaHS has higher solubility (≥ 500 g/L at 25°C) compared to Na₂S·9H₂O (154 g/L at 10°C), but impurities in commercial NaHS salts often complicate experimental reproducibility .
- Safety : Both compounds are corrosive and toxic, but Na₂S·9H₂O’s solid form simplifies handling compared to NaHS powders .
Sodium Sulfate Decahydrate (Na₂SO₄·10H₂O)
- Thermochemical Storage : While Na₂S·9H₂O exhibits high energy density (1.2–1.5 GJ/m³) for heat storage, Na₂SO₄·10H₂O has lower enthalpy changes (~0.3 GJ/m³) but superior mechanical stability during hydration-dehydration cycles .
- Environmental Impact: Na₂S·9H₂O poses higher aquatic toxicity (WGK Germany class 2) compared to Na₂SO₄·10H₂O, which is generally regarded as non-toxic .
Organic H₂S Donors (e.g., Diallyl Trisulfide, DATS)
- Release Kinetics: Garlic-derived donors like DATS release H₂S slowly via enzymatic cleavage, offering sustained biological effects compared to the instantaneous release from Na₂S·9H₂O .
- Clinical Potential: DATS and similar organosulfur compounds show promise in cardiovascular therapies due to their prolonged H₂S release, whereas Na₂S·9H₂O’s rapid dissociation limits its therapeutic window .
Table 1: Comparative Properties of Sodium Sulfide Nonahydrate and Related Compounds
Property | Na₂S·9H₂O | Na₂S (Anhydrous) | NaHS | Na₂SO₄·10H₂O | DATS |
---|---|---|---|---|---|
Formula | Na₂S·9H₂O | Na₂S | NaHS | Na₂SO₄·10H₂O | C₆H₁₀S₃ |
H₂S Release | Rapid, pH-dependent | Rapid, moisture-sensitive | Immediate | None | Slow, enzyme-dependent |
Solubility (H₂O) | 154 g/L (10°C) | Reacts violently | ≥500 g/L (25°C) | 44 g/100 mL (0°C) | Insoluble |
Thermal Stability | Dehydrates above 50°C | Stable if anhydrous | Stable | Stable up to 32.4°C | Thermally stable |
Key Applications | Organic synthesis, H₂S generation | Limited industrial use | Lab-scale H₂S studies | Heat storage, detergents | Cardiovascular research |
Toxicity | Acute toxicity (Oral LD₅₀: 300 mg/kg) | Similar to nonahydrate | Corrosive, toxic | Low | Low |
References |
Preparation Methods
Suspension Cooling Crystallization
Methodology and Process Parameters
Suspension cooling crystallization is a widely employed technique for producing high-purity Na₂S·9H₂O crystals. As described by Roelands et al. , the process involves dissolving sodium sulfide lumps in deionized water at elevated temperatures (60–80°C) to form a saturated solution. The solution is then cooled to room temperature (20–25°C) at a controlled rate of 1–2°C/min, inducing supersaturation and subsequent crystallization. The nonahydrate phase is selectively formed by maintaining the temperature below 49°C, as higher temperatures favor the pentahydrate (Na₂S·5H₂O) or anhydrous forms .
Key Steps:
-
Dissolution : Na₂S lumps are dissolved in water at 80°C to achieve a 40–50% (w/w) concentration.
-
Cooling : The solution is cooled to 20°C with continuous stirring to ensure uniform crystal growth.
-
Filtration : Crystals are vacuum-filtered and washed with cold ethanol to remove residual impurities.
Stabilization with Cellulose:
To mitigate phase instability during thermal cycling, cellulose fibers (5–10% w/w) are dispersed in the hot solution before cooling. This composite material prevents agglomeration and maintains structural integrity over multiple hydration-dehydration cycles .
Reaction of Elemental Sulfur with Sodium Hydroxide
Stoichiometric Synthesis
This method involves the direct reaction of elemental sulfur (S₈) with sodium hydroxide (NaOH) in aqueous medium, as outlined by Sigma-Aldrich :
The reaction is conducted at 80–90°C under inert atmosphere to minimize oxidation. Excess water is then added to crystallize Na₂S·9H₂O, which is isolated via fractional crystallization .
Optimization Conditions:
-
Molar Ratio : 1:1.5 (S:NaOH) to maximize Na₂S yield.
-
Crystallization Temperature : 10–15°C to favor nonahydrate formation.
-
Purity Control : Residual polysulfides (Na₂Sₓ, x > 1) are removed by washing with ethanol .
Gas-Phase Sulfidation Using Hydrogen Sulfide
Laboratory-Scale Synthesis
A video demonstration by PoorMansChemist5 details the generation of H₂S gas via the reaction of ferrous sulfide (FeS) with sulfuric acid (H₂SO₄):
The H₂S gas is bubbled into a chilled NaOH solution (2–4 M), resulting in the precipitation of Na₂S·9H₂O:
Critical Considerations:
-
Gas Purification : H₂S is passed through a condenser at 7°C to remove elemental sulfur impurities5.
-
Neutralization Efficiency : Excess NaOH (pH > 12) ensures complete conversion to Na₂S .
Carbothermic Reduction of Sodium Sulfate (Industrial Method)
High-Temperature Reduction
The industrial production of Na₂S·9H₂O involves carbothermic reduction of sodium sulfate (Na₂SO₄) at 900–1200°C :
The resultant anhydrous Na₂S is hydrated in a controlled humidity chamber (RH > 80%) to form the nonahydrate.
Process Metrics:
Recrystallization from Lower Hydrates
Hydration of Anhydrous or Pentahydrate Forms
Na₂S·9H₂O can be synthesized by hydrating lower hydrates (e.g., Na₂S·5H₂O) or anhydrous Na₂S. A patent by Alig describes dissolving Na₂S·5H₂O in water at 40°C and cooling to 20°C under vacuum (50–100 mbar) to induce nonahydrate crystallization.
Phase Diagram Guidance:
Microwave-Assisted Synthesis
Rapid Hydration Technique
Although primarily used for anhydrous Na₂S , microwave-assisted synthesis can be adapted for Na₂S·9H₂O by irradiating a Na₂S solution (60–70°C) with 2.45 GHz microwaves for 5–10 minutes, followed by rapid quenching in an ice bath.
Advantages:
Comparative Analysis of Methods
Properties
Molecular Formula |
H18Na2O9S |
---|---|
Molecular Weight |
240.19 g/mol |
IUPAC Name |
disodium;sulfide;nonahydrate |
InChI |
InChI=1S/2Na.9H2O.S/h;;9*1H2;/q2*+1;;;;;;;;;;-2 |
InChI Key |
ZGHLCBJZQLNUAZ-UHFFFAOYSA-N |
SMILES |
O.O.O.O.O.O.O.O.O.[Na+].[Na+].[S-2] |
Canonical SMILES |
O.O.O.O.O.O.O.O.O.[Na+].[Na+].[S-2] |
Synonyms |
Actisoufre Na2S natrum sulphuratum sodium monosulfide sodium monosulfide nonahydrate sodium monosulphide sodium monosulphide nonahydrate sodium sulfide sodium sulfide anhydrous sodium sulfide nonahydrate sodium sulphide anhydrous |
Origin of Product |
United States |
Retrosynthesis Analysis
AI-Powered Synthesis Planning: Our tool employs the Template_relevance Pistachio, Template_relevance Bkms_metabolic, Template_relevance Pistachio_ringbreaker, Template_relevance Reaxys, Template_relevance Reaxys_biocatalysis model, leveraging a vast database of chemical reactions to predict feasible synthetic routes.
One-Step Synthesis Focus: Specifically designed for one-step synthesis, it provides concise and direct routes for your target compounds, streamlining the synthesis process.
Accurate Predictions: Utilizing the extensive PISTACHIO, BKMS_METABOLIC, PISTACHIO_RINGBREAKER, REAXYS, REAXYS_BIOCATALYSIS database, our tool offers high-accuracy predictions, reflecting the latest in chemical research and data.
Strategy Settings
Precursor scoring | Relevance Heuristic |
---|---|
Min. plausibility | 0.01 |
Model | Template_relevance |
Template Set | Pistachio/Bkms_metabolic/Pistachio_ringbreaker/Reaxys/Reaxys_biocatalysis |
Top-N result to add to graph | 6 |
Feasible Synthetic Routes
Disclaimer and Information on In-Vitro Research Products
Please be aware that all articles and product information presented on BenchChem are intended solely for informational purposes. The products available for purchase on BenchChem are specifically designed for in-vitro studies, which are conducted outside of living organisms. In-vitro studies, derived from the Latin term "in glass," involve experiments performed in controlled laboratory settings using cells or tissues. It is important to note that these products are not categorized as medicines or drugs, and they have not received approval from the FDA for the prevention, treatment, or cure of any medical condition, ailment, or disease. We must emphasize that any form of bodily introduction of these products into humans or animals is strictly prohibited by law. It is essential to adhere to these guidelines to ensure compliance with legal and ethical standards in research and experimentation.