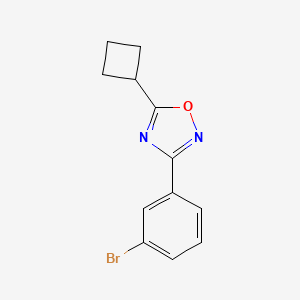
3-(3-Bromophenyl)-5-cyclobutyl-1,2,4-oxadiazole
- Click on QUICK INQUIRY to receive a quote from our team of experts.
- With the quality product at a COMPETITIVE price, you can focus more on your research.
Overview
Description
3-(3-Bromophenyl)-5-cyclobutyl-1,2,4-oxadiazole is a heterocyclic compound that contains a bromophenyl group and a cyclobutyl group attached to an oxadiazole ring
Preparation Methods
Synthetic Routes and Reaction Conditions
The synthesis of 3-(3-Bromophenyl)-5-cyclobutyl-1,2,4-oxadiazole typically involves the cyclization of appropriate precursors under specific conditions. One common method involves the reaction of 3-bromobenzohydrazide with cyclobutanone in the presence of a dehydrating agent such as phosphorus oxychloride (POCl3). The reaction is carried out under reflux conditions, leading to the formation of the oxadiazole ring.
Industrial Production Methods
Industrial production of this compound may involve similar synthetic routes but on a larger scale. The use of continuous flow reactors and optimized reaction conditions can enhance the efficiency and yield of the process. Additionally, purification techniques such as recrystallization and chromatography are employed to obtain the desired product with high purity.
Chemical Reactions Analysis
Types of Reactions
3-(3-Bromophenyl)-5-cyclobutyl-1,2,4-oxadiazole undergoes various chemical reactions, including:
Substitution Reactions: The bromine atom on the phenyl ring can be substituted with other functional groups through reactions such as Suzuki-Miyaura coupling.
Oxidation and Reduction: The compound can undergo oxidation and reduction reactions, leading to the formation of different derivatives.
Cyclization Reactions: The oxadiazole ring can participate in cyclization reactions to form more complex heterocyclic structures.
Common Reagents and Conditions
Suzuki-Miyaura Coupling: This reaction involves the use of palladium catalysts and boronic acids to substitute the bromine atom with various functional groups.
Oxidizing Agents: Reagents such as potassium permanganate (KMnO4) or chromium trioxide (CrO3) can be used for oxidation reactions.
Reducing Agents: Sodium borohydride (NaBH4) or lithium aluminum hydride (LiAlH4) are commonly used for reduction reactions.
Major Products
The major products formed from these reactions depend on the specific reagents and conditions used. For example, Suzuki-Miyaura coupling can yield various substituted phenyl derivatives, while oxidation and reduction reactions can lead to the formation of different oxadiazole derivatives.
Scientific Research Applications
3-(3-Bromophenyl)-5-cyclobutyl-1,2,4-oxadiazole has several scientific research applications:
Medicinal Chemistry: The compound is studied for its potential as a pharmacophore in drug design, particularly for its ability to interact with biological targets.
Materials Science: Its unique structural features make it a candidate for the development of novel materials with specific electronic and optical properties.
Biological Studies: The compound is used in biological assays to study its effects on various cellular processes and pathways.
Industrial Applications: It can be used as an intermediate in the synthesis of more complex molecules for industrial purposes.
Mechanism of Action
The mechanism of action of 3-(3-Bromophenyl)-5-cyclobutyl-1,2,4-oxadiazole involves its interaction with specific molecular targets. The bromophenyl group can engage in π-π stacking interactions with aromatic residues in proteins, while the oxadiazole ring can form hydrogen bonds with amino acid side chains. These interactions can modulate the activity of enzymes and receptors, leading to various biological effects.
Comparison with Similar Compounds
Similar Compounds
3-(3-Bromophenyl)-1,2,4-triazole: Similar in structure but contains a triazole ring instead of an oxadiazole ring.
3-(3-Bromophenyl)-5-methyl-1,2,4-oxadiazole: Contains a methyl group instead of a cyclobutyl group.
3-(3-Bromophenyl)-1,2,4-thiadiazole: Contains a thiadiazole ring instead of an oxadiazole ring.
Uniqueness
3-(3-Bromophenyl)-5-cyclobutyl-1,2,4-oxadiazole is unique due to the presence of both a cyclobutyl group and an oxadiazole ring. This combination imparts distinct electronic and steric properties, making it a valuable compound for various applications in research and industry.
Properties
Molecular Formula |
C12H11BrN2O |
---|---|
Molecular Weight |
279.13 g/mol |
IUPAC Name |
3-(3-bromophenyl)-5-cyclobutyl-1,2,4-oxadiazole |
InChI |
InChI=1S/C12H11BrN2O/c13-10-6-2-5-9(7-10)11-14-12(16-15-11)8-3-1-4-8/h2,5-8H,1,3-4H2 |
InChI Key |
AELQNKVTTBXYRB-UHFFFAOYSA-N |
Canonical SMILES |
C1CC(C1)C2=NC(=NO2)C3=CC(=CC=C3)Br |
Origin of Product |
United States |
Disclaimer and Information on In-Vitro Research Products
Please be aware that all articles and product information presented on BenchChem are intended solely for informational purposes. The products available for purchase on BenchChem are specifically designed for in-vitro studies, which are conducted outside of living organisms. In-vitro studies, derived from the Latin term "in glass," involve experiments performed in controlled laboratory settings using cells or tissues. It is important to note that these products are not categorized as medicines or drugs, and they have not received approval from the FDA for the prevention, treatment, or cure of any medical condition, ailment, or disease. We must emphasize that any form of bodily introduction of these products into humans or animals is strictly prohibited by law. It is essential to adhere to these guidelines to ensure compliance with legal and ethical standards in research and experimentation.