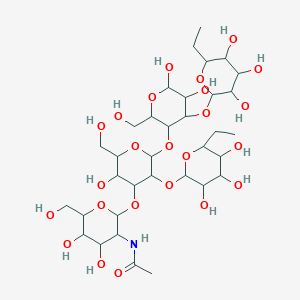
A-Pentasaccharide
- Click on QUICK INQUIRY to receive a quote from our team of experts.
- With the quality product at a COMPETITIVE price, you can focus more on your research.
Overview
Description
A-Pentasaccharide is a complex carbohydrate composed of five monosaccharide units. It is a significant compound in various biological processes and has been studied for its potential therapeutic applications. The structure of this compound allows it to interact with specific proteins and receptors, making it a valuable molecule in medicinal chemistry and drug design.
Preparation Methods
Synthetic Routes and Reaction Conditions
The synthesis of A-Pentasaccharide typically involves a series of glycosylation reactions. These reactions are carried out under controlled conditions to ensure the correct stereochemistry of the glycosidic bonds. Commonly used methods include the use of glycosyl donors and acceptors, with catalysts such as Lewis acids or enzymes to facilitate the reaction .
Industrial Production Methods
Industrial production of this compound can be achieved through chemoenzymatic synthesis. This method combines chemical synthesis with enzymatic steps to produce the desired pentasaccharide with high yield and purity. The process involves the use of specific enzymes that catalyze the formation of glycosidic bonds, followed by purification steps to isolate the final product .
Chemical Reactions Analysis
Types of Reactions
A-Pentasaccharide undergoes various chemical reactions, including:
Reduction: This reaction involves the addition of hydrogen atoms, leading to the formation of alcohols or alkanes.
Substitution: This reaction involves the replacement of one functional group with another, such as the substitution of hydroxyl groups with halogens.
Common Reagents and Conditions
Common reagents used in these reactions include oxidizing agents like potassium permanganate, reducing agents like sodium borohydride, and halogenating agents like thionyl chloride. The reactions are typically carried out under controlled temperatures and pH conditions to ensure the desired outcome .
Major Products Formed
The major products formed from these reactions depend on the specific conditions and reagents used. For example, oxidation of this compound can lead to the formation of uronic acids, while reduction can produce alditols. Substitution reactions can yield various derivatives with modified functional groups .
Scientific Research Applications
A-Pentasaccharide has a wide range of applications in scientific research:
Chemistry: It is used as a building block for the synthesis of more complex carbohydrates and glycoconjugates.
Biology: It plays a role in cell signaling and recognition processes, making it a valuable tool for studying cellular interactions.
Medicine: This compound has been investigated for its potential as an anticoagulant, antiviral, and anti-inflammatory agent
Industry: It is used in the production of vaccines and as a component in drug delivery systems.
Mechanism of Action
The mechanism of action of A-Pentasaccharide involves its interaction with specific molecular targets, such as proteins and receptors. For example, in its role as an anticoagulant, this compound binds to antithrombin and enhances its inhibitory effect on factor Xa, preventing blood clot formation . The molecular pathways involved in these interactions are complex and often involve multiple steps, including binding, conformational changes, and signal transduction .
Comparison with Similar Compounds
A-Pentasaccharide can be compared with other similar compounds, such as:
Heparin: A widely used anticoagulant that also interacts with antithrombin but has a more complex structure and higher molecular weight.
Fondaparinux: A synthetic pentasaccharide that specifically inhibits factor Xa and has a more predictable pharmacokinetic profile compared to heparin.
Oligosaccharides: These are shorter or longer chains of monosaccharides that can have similar or different biological activities depending on their structure.
The uniqueness of this compound lies in its specific structure, which allows for targeted interactions with molecular targets, making it a valuable compound in both research and therapeutic applications .
Properties
Molecular Formula |
C34H59NO24 |
---|---|
Molecular Weight |
865.8 g/mol |
IUPAC Name |
N-[2-[3-(6-ethyl-3,4,5-trihydroxyoxan-2-yl)oxy-2-[4-(6-ethyl-3,4,5-trihydroxyoxan-2-yl)oxy-5,6-dihydroxy-2-(hydroxymethyl)oxan-3-yl]oxy-5-hydroxy-6-(hydroxymethyl)oxan-4-yl]oxy-4,5-dihydroxy-6-(hydroxymethyl)oxan-3-yl]acetamide |
InChI |
InChI=1S/C34H59NO24/c1-4-10-16(40)21(45)23(47)32(52-10)58-28-25(49)30(50)51-14(8-38)26(28)56-34-29(59-33-24(48)22(46)17(41)11(5-2)53-33)27(19(43)13(7-37)55-34)57-31-15(35-9(3)39)20(44)18(42)12(6-36)54-31/h10-34,36-38,40-50H,4-8H2,1-3H3,(H,35,39) |
InChI Key |
SNSSOBJOMCQZRC-UHFFFAOYSA-N |
Canonical SMILES |
CCC1C(C(C(C(O1)OC2C(C(OC(C2OC3C(C(C(C(O3)CO)O)OC4C(C(C(C(O4)CO)O)O)NC(=O)C)OC5C(C(C(C(O5)CC)O)O)O)CO)O)O)O)O)O |
Origin of Product |
United States |
Disclaimer and Information on In-Vitro Research Products
Please be aware that all articles and product information presented on BenchChem are intended solely for informational purposes. The products available for purchase on BenchChem are specifically designed for in-vitro studies, which are conducted outside of living organisms. In-vitro studies, derived from the Latin term "in glass," involve experiments performed in controlled laboratory settings using cells or tissues. It is important to note that these products are not categorized as medicines or drugs, and they have not received approval from the FDA for the prevention, treatment, or cure of any medical condition, ailment, or disease. We must emphasize that any form of bodily introduction of these products into humans or animals is strictly prohibited by law. It is essential to adhere to these guidelines to ensure compliance with legal and ethical standards in research and experimentation.