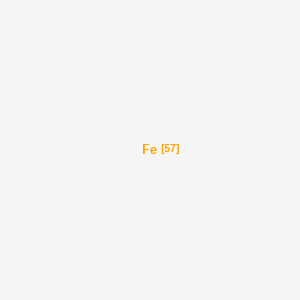
Iron, isotope of mass 57
Overview
Description
Iron-57 is a stable isotope of Iron . It has a mass number of 57, which means it has 57 nucleons (protons and neutrons combined). The atomic number of Iron is 26, which means Iron-57 has 26 protons and 31 neutrons . The isotopic mass of Iron-57 is 56.935393 atomic units . It is both naturally occurring and produced by fission .
Synthesis Analysis
Iron-57 is naturally occurring and also produced by fission . It is used in Mössbauer spectroscopy, and Co-60 sources are used for the measurements . From these sources, Iron-57 nuclei are formed by electron capture with a half-life of 280 days, which in turn gradually decay with loss of energy .
Molecular Structure Analysis
Iron-57 has a nuclear magnetic moment of +0.09064 and a gyromagnetic ratio of 0.8661 x 10^7 rad T^-1 s^-1 . It has a nuclear g-factor of 0.18128 and a quadrupole moment of +0.160 barn . The resonance frequency of Iron-57 is 1.3816 at 1 Tesla .
Chemical Reactions Analysis
Iron is a very active metal and readily combines with oxygen in moist air . The product of this reaction, iron oxide (Fe2O3), is known as rust . Iron also reacts with very hot water and steam to produce hydrogen gas . It also dissolves in most acids and reacts with many other elements .
Physical And Chemical Properties Analysis
Iron-57 has a nuclear binding energy of 499.90509736 MeV per nucleus . It has a charge radius of 3.7532 femtometers . The isotopic mass of Iron-57 is 56.935393 atomic units, and the nuclide mass is 56.9211303 atomic units .
Scientific Research Applications
Mössbauer Spectroscopy
Iron-57 is widely used in Mössbauer spectroscopy , a technique used to study the resonance absorption of gamma rays . This method is particularly useful for investigating the properties of iron-containing compounds and materials .
Characterization of Silicate Glasses
Iron-57 has been used in the characterization of silicate glasses used for high temperature incinerated low-level radioactive waste products . The iron-57 Mössbauer spectroscopy was used to investigate the structure of these glasses .
Investigation of Iron Alloys
Iron-57 has been used to study the hyperfine magnetic field distributions in iron alloys . This application provides valuable insights into the properties and behavior of these alloys .
Study of Radioactive Waste Products
Iron-57 has been applied in the study of radioactive waste products . The Mössbauer spectroscopy of iron-57 provides valuable information about the structure and composition of these waste products .
Investigation of Iron (II) and Iron (III) Glasses
Iron-57 has been used to investigate the properties of iron (II) and iron (III) glasses . The Mössbauer spectroscopy of iron-57 provides insights into the structure and composition of these glasses .
Study of Crystallization Process
Iron-57 has been used to study the crystallization process in certain materials . The Mössbauer spectroscopy of iron-57 provides valuable information about the influence of composition and quenching mode upon the crystallization process .
Future Directions
Iron isotopes, including Iron-57, have been actively measured since 1999 and have been used to track a wide range of both high- and low-temperature geochemical, as well as cosmochemical processes . The isotopic signature of Fe is particularly sensitive to redox effects, including certain biological reactions such as bacterial dissimilatory iron reduction (DIR) . This work has been driven by advances in mass spectrometry technology . Future research may continue to explore the use of iron isotopes in various fields, including environmental science, geology, and astrobiology .
properties
IUPAC Name |
iron-57 | |
---|---|---|
Source | PubChem | |
URL | https://pubchem.ncbi.nlm.nih.gov | |
Description | Data deposited in or computed by PubChem | |
InChI |
InChI=1S/Fe/i1+1 | |
Source | PubChem | |
URL | https://pubchem.ncbi.nlm.nih.gov | |
Description | Data deposited in or computed by PubChem | |
InChI Key |
XEEYBQQBJWHFJM-OUBTZVSYSA-N | |
Source | PubChem | |
URL | https://pubchem.ncbi.nlm.nih.gov | |
Description | Data deposited in or computed by PubChem | |
Canonical SMILES |
[Fe] | |
Source | PubChem | |
URL | https://pubchem.ncbi.nlm.nih.gov | |
Description | Data deposited in or computed by PubChem | |
Isomeric SMILES |
[57Fe] | |
Source | PubChem | |
URL | https://pubchem.ncbi.nlm.nih.gov | |
Description | Data deposited in or computed by PubChem | |
Molecular Formula |
Fe | |
Source | PubChem | |
URL | https://pubchem.ncbi.nlm.nih.gov | |
Description | Data deposited in or computed by PubChem | |
DSSTOX Substance ID |
DTXSID60933168 | |
Record name | (~57~Fe)Iron | |
Source | EPA DSSTox | |
URL | https://comptox.epa.gov/dashboard/DTXSID60933168 | |
Description | DSSTox provides a high quality public chemistry resource for supporting improved predictive toxicology. | |
Molecular Weight |
56.935392 g/mol | |
Source | PubChem | |
URL | https://pubchem.ncbi.nlm.nih.gov | |
Description | Data deposited in or computed by PubChem | |
Product Name |
Iron, isotope of mass 57 | |
CAS RN |
14762-69-7 | |
Record name | Iron, isotope of mass 57 | |
Source | ChemIDplus | |
URL | https://pubchem.ncbi.nlm.nih.gov/substance/?source=chemidplus&sourceid=0014762697 | |
Description | ChemIDplus is a free, web search system that provides access to the structure and nomenclature authority files used for the identification of chemical substances cited in National Library of Medicine (NLM) databases, including the TOXNET system. | |
Record name | (~57~Fe)Iron | |
Source | EPA DSSTox | |
URL | https://comptox.epa.gov/dashboard/DTXSID60933168 | |
Description | DSSTox provides a high quality public chemistry resource for supporting improved predictive toxicology. | |
Synthesis routes and methods I
Procedure details
Synthesis routes and methods II
Procedure details
Synthesis routes and methods III
Procedure details
Q & A
Q1: What makes iron-57 particularly suitable for Mössbauer spectroscopy?
A: Iron-57 exhibits a phenomenon called the Mössbauer effect, where it can resonantly absorb and emit gamma rays without significant energy loss due to recoil. This property makes it an exceptional probe for investigating the electronic structure, magnetic properties, and local environments of iron-containing materials. []
Q2: How does iron-57 Mössbauer spectroscopy contribute to understanding magnetization dynamics?
A: It provides insights into magnetization dynamics on a significantly faster timescale compared to conventional methods like AC magnetometry. This capability is attributed to the short lifetime of the iron-57 excited state (10-8 to 10-9 seconds). Studies on linear iron(I) and iron(II) complexes have demonstrated the effectiveness of iron-57 Mössbauer spectroscopy in revealing Orbach relaxation processes and temperature-dependent magnetic relaxation mechanisms. []
Q3: What is the molecular formula and weight of iron-57?
A3: Iron-57, being an isotope of iron, doesn't have a distinct molecular formula. Its isotopic symbol is 57Fe, indicating 26 protons and 31 neutrons. Its atomic weight is approximately 56.9354 atomic mass units.
Q4: How does iron-57 Mössbauer spectroscopy help determine the oxidation state of iron in a sample?
A: The isomer shift (δ) obtained from iron-57 Mössbauer spectra is sensitive to the s-electron density at the iron nucleus. This density is influenced by the oxidation state of iron; for example, Fe(II) generally exhibits a larger positive isomer shift compared to Fe(III) due to greater shielding of the nucleus by d-electrons in the +2 oxidation state. []
Q5: What information can be obtained from the quadrupole splitting in an iron-57 Mössbauer spectrum?
A: Quadrupole splitting (ΔEQ) arises from the interaction between the electric quadrupole moment of the iron-57 nucleus and the electric field gradient (EFG) at the nucleus. It provides insights into the symmetry and electronic environment around the iron atom, helping determine factors like coordination geometry, ligand field strength, and the presence of nearby defects. []
Q6: How does the cooling rate affect the magnetic structure of NdMn6−xFexSn6 compounds as studied by iron-57 Mössbauer spectroscopy?
A: Iron-57 and tin-119 Mössbauer spectra revealed that the spin reorientation in these compounds is highly sensitive to the cooling rate. Slowly cooled samples retain their high-temperature magnetic structure, while quenched samples exhibit spin reorientation at low temperatures. This sensitivity highlights the importance of controlled thermal treatments in materials processing and characterization. []
Q7: How is iron-57 Mössbauer spectroscopy applied in the study of corrosion?
A: Iron-57 Mössbauer spectroscopy is a valuable tool in corrosion science. It allows for the identification and quantification of various iron oxides and oxyhydroxides, which are common corrosion products. This information helps determine the corrosion mechanism, the nature of the corrosive environment, and the effectiveness of corrosion inhibitors. []
Q8: What are the applications of iron-57 Mössbauer spectroscopy in catalysis research?
A: This technique is widely employed to characterize catalysts, providing information about the oxidation state, coordination environment, and interactions between the active metal sites and the support material. This knowledge is crucial for understanding catalytic mechanisms, optimizing catalyst performance, and designing new catalytic materials. []
Q9: How can iron-57 Mössbauer spectroscopy be used to study biological systems?
A: It provides valuable information about the iron-containing active sites in proteins like hemoglobin, myoglobin, and cytochromes. By analyzing the isomer shift and quadrupole splitting, researchers can deduce the spin state, coordination geometry, and nature of the ligands surrounding the iron center, contributing to a deeper understanding of these biomolecules' structure and function. [] []
Q10: How is computational chemistry used in conjunction with iron-57 Mössbauer spectroscopy?
A: Density functional theory (DFT) calculations are often coupled with iron-57 Mössbauer spectroscopy to gain deeper insights into electronic structure and bonding. DFT can predict Mössbauer parameters like isomer shift and quadrupole splitting, which can be directly compared to experimental data to validate structural models and understand the factors influencing hyperfine interactions. [] []
Q11: How is iron-57 Mössbauer spectroscopy used in studying nanomaterials?
A: It is a powerful tool for characterizing iron-containing nanomaterials. It provides information about the particle size, size distribution, magnetic properties, and surface effects, which are crucial for understanding the unique properties of nanomaterials and their potential applications. []
Disclaimer and Information on In-Vitro Research Products
Please be aware that all articles and product information presented on BenchChem are intended solely for informational purposes. The products available for purchase on BenchChem are specifically designed for in-vitro studies, which are conducted outside of living organisms. In-vitro studies, derived from the Latin term "in glass," involve experiments performed in controlled laboratory settings using cells or tissues. It is important to note that these products are not categorized as medicines or drugs, and they have not received approval from the FDA for the prevention, treatment, or cure of any medical condition, ailment, or disease. We must emphasize that any form of bodily introduction of these products into humans or animals is strictly prohibited by law. It is essential to adhere to these guidelines to ensure compliance with legal and ethical standards in research and experimentation.