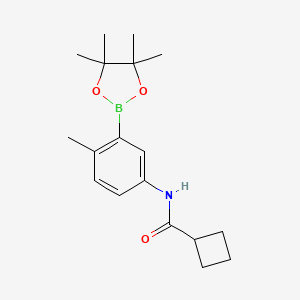
N-(4-methyl-3-(4,4,5,5-tetramethyl-1,3,2-dioxaborolan-2-yl)phenyl)cyclobutanecarboxamide
- Click on QUICK INQUIRY to receive a quote from our team of experts.
- With the quality product at a COMPETITIVE price, you can focus more on your research.
Overview
Description
N-(4-methyl-3-(4,4,5,5-tetramethyl-1,3,2-dioxaborolan-2-yl)phenyl)cyclobutanecarboxamide is a complex organic compound that features a cyclobutanecarboxamide group attached to a phenyl ring, which is further substituted with a dioxaborolane moiety
Preparation Methods
Synthetic Routes and Reaction Conditions
The synthesis of N-(4-methyl-3-(4,4,5,5-tetramethyl-1,3,2-dioxaborolan-2-yl)phenyl)cyclobutanecarboxamide typically involves multiple steps. One common method includes the Suzuki-Miyaura coupling reaction, where a boronic acid derivative reacts with an aryl halide in the presence of a palladium catalyst . The reaction conditions often require a base such as potassium carbonate and a solvent like toluene or ethanol.
Industrial Production Methods
Industrial production of this compound may involve similar synthetic routes but on a larger scale. The process is optimized for higher yields and purity, often using automated systems for precise control of reaction parameters. The use of continuous flow reactors can enhance the efficiency and scalability of the production process .
Chemical Reactions Analysis
Types of Reactions
N-(4-methyl-3-(4,4,5,5-tetramethyl-1,3,2-dioxaborolan-2-yl)phenyl)cyclobutanecarboxamide can undergo various chemical reactions, including:
Oxidation: The compound can be oxidized using reagents like hydrogen peroxide or potassium permanganate.
Reduction: Reduction reactions can be carried out using agents such as lithium aluminum hydride.
Substitution: The dioxaborolane moiety can participate in substitution reactions, particularly in the presence of nucleophiles.
Common Reagents and Conditions
Oxidation: Hydrogen peroxide in an aqueous medium.
Reduction: Lithium aluminum hydride in anhydrous ether.
Substitution: Nucleophiles like amines or alcohols in the presence of a base.
Major Products
The major products formed from these reactions depend on the specific conditions and reagents used. For example, oxidation may yield a corresponding carboxylic acid, while reduction could produce an alcohol derivative .
Scientific Research Applications
N-(4-methyl-3-(4,4,5,5-tetramethyl-1,3,2-dioxaborolan-2-yl)phenyl)cyclobutanecarboxamide has several applications in scientific research:
Biology: Investigated for its potential as a biochemical probe due to its ability to interact with specific biomolecules.
Industry: Utilized in the development of advanced materials, such as polymers and nanomaterials.
Mechanism of Action
The mechanism by which N-(4-methyl-3-(4,4,5,5-tetramethyl-1,3,2-dioxaborolan-2-yl)phenyl)cyclobutanecarboxamide exerts its effects involves its interaction with molecular targets such as enzymes or receptors. The dioxaborolane moiety can form reversible covalent bonds with nucleophilic sites on proteins, modulating their activity and leading to various biological effects .
Comparison with Similar Compounds
Similar Compounds
- N,N-Dimethyl-4-(4,4,5,5-tetramethyl-1,3,2-dioxaborolan-2-yl)aniline
- 1-Methylpyrazole-4-boronic acid pinacol ester
Uniqueness
N-(4-methyl-3-(4,4,5,5-tetramethyl-1,3,2-dioxaborolan-2-yl)phenyl)cyclobutanecarboxamide is unique due to its combination of a cyclobutanecarboxamide group with a dioxaborolane moiety, which imparts distinct chemical reactivity and potential for diverse applications .
Properties
Molecular Formula |
C18H26BNO3 |
---|---|
Molecular Weight |
315.2 g/mol |
IUPAC Name |
N-[4-methyl-3-(4,4,5,5-tetramethyl-1,3,2-dioxaborolan-2-yl)phenyl]cyclobutanecarboxamide |
InChI |
InChI=1S/C18H26BNO3/c1-12-9-10-14(20-16(21)13-7-6-8-13)11-15(12)19-22-17(2,3)18(4,5)23-19/h9-11,13H,6-8H2,1-5H3,(H,20,21) |
InChI Key |
XSEYGVRNPIEKRX-UHFFFAOYSA-N |
Canonical SMILES |
B1(OC(C(O1)(C)C)(C)C)C2=C(C=CC(=C2)NC(=O)C3CCC3)C |
Origin of Product |
United States |
Disclaimer and Information on In-Vitro Research Products
Please be aware that all articles and product information presented on BenchChem are intended solely for informational purposes. The products available for purchase on BenchChem are specifically designed for in-vitro studies, which are conducted outside of living organisms. In-vitro studies, derived from the Latin term "in glass," involve experiments performed in controlled laboratory settings using cells or tissues. It is important to note that these products are not categorized as medicines or drugs, and they have not received approval from the FDA for the prevention, treatment, or cure of any medical condition, ailment, or disease. We must emphasize that any form of bodily introduction of these products into humans or animals is strictly prohibited by law. It is essential to adhere to these guidelines to ensure compliance with legal and ethical standards in research and experimentation.