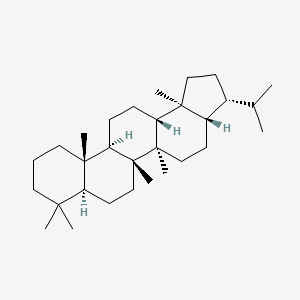
Hopane
Overview
Description
Hopane is a natural chemical compound classified as a triterpeneThe first compound of the this compound family to be isolated and characterized was hydroxyhopanone, found in dammar resin . This compound is a pentacyclic triterpene, meaning it has five interconnected rings in its structure. It is widely distributed in nature and is found in the cell membranes of various bacteria, lichens, bryophytes, ferns, tropical trees, and fungi .
Mechanism of Action
Target of Action
Hopane, also known as A’-Neogammacerane, is a natural chemical compound classified as a triterpene . It forms the central core of a variety of other chemical compounds collectively known as hopanoids . The primary targets of this compound are Squalene Hopene Cyclases (SHC) . SHCs convert squalene, a linear triterpene, into a fused ring product called hopanoid .
Mode of Action
This compound interacts with its targets, the SHCs, through a cationic cyclization mechanism . This interaction results in the conversion of squalene into hopanoid . The SHCs are capable of accommodating substrates other than their natural substrate, which can be exploited for industrial applications .
Biochemical Pathways
The biochemical pathway primarily affected by this compound is the cyclization of squalene into hopanoids . This process involves the formation of five ring structures, thirteen covalent bonds, and nine stereo centers . The cascade of cationic cyclization reactions catalyzed by SHCs in the conversion of squalene to hopanoids imparts structural stability to biological membranes .
Result of Action
The primary result of this compound’s action is the production of hopanoids, a class of pentacyclic triterpenoids . These hopanoids play a crucial role in maintaining membrane fluidity and stability in bacteria . This can have significant effects at the molecular and cellular levels, influencing the overall function and stability of the cell.
Action Environment
The action of this compound can be influenced by various environmental factors. For instance, hopanoid-producing bacteria are abundant in various ecosystems, such as the rhizosphere . The production of hopanoids can affect bacterial interactions with other organisms, such as plants . .
Biochemical Analysis
Biochemical Properties
Hopane is involved in several biochemical reactions within bacterial cells. It interacts with various enzymes, proteins, and other biomolecules to maintain membrane integrity and function. One of the key enzymes involved in this compound biosynthesis is squalene-hopene cyclase, which catalyzes the cyclization of squalene to form this compound. This enzyme is crucial for the production of hopanoids, which are essential for the stability and functionality of bacterial membranes .
Cellular Effects
This compound influences various cellular processes, including cell signaling pathways, gene expression, and cellular metabolism. In bacterial cells, this compound and its derivatives help maintain membrane fluidity, which is essential for proper cell function. They also play a role in protecting cells from environmental stressors, such as changes in temperature and pH. Additionally, this compound can influence the expression of genes involved in lipid metabolism and membrane synthesis .
Molecular Mechanism
At the molecular level, this compound exerts its effects through interactions with membrane lipids and proteins. It can bind to specific sites on membrane proteins, modulating their activity and function. This compound also affects the organization of lipid bilayers, enhancing membrane stability and reducing permeability. These interactions are crucial for maintaining the structural integrity of bacterial membranes and ensuring proper cellular function .
Temporal Effects in Laboratory Settings
In laboratory settings, the effects of this compound can change over time due to its stability and degradation. This compound is relatively stable under various conditions, but it can degrade over time, leading to changes in its effects on cellular function. Long-term studies have shown that this compound can have lasting effects on membrane stability and cell viability, even after prolonged exposure .
Dosage Effects in Animal Models
The effects of this compound can vary with different dosages in animal models. At low doses, this compound may enhance membrane stability and protect cells from environmental stressors. At high doses, this compound can have toxic effects, leading to cell damage and impaired function. Threshold effects have been observed, where the beneficial effects of this compound are only seen within a specific dosage range .
Metabolic Pathways
This compound is involved in several metabolic pathways within bacterial cells. It interacts with enzymes and cofactors involved in lipid metabolism, influencing the synthesis and degradation of membrane lipids. This compound can also affect metabolic flux, altering the levels of various metabolites within the cell. These interactions are crucial for maintaining cellular homeostasis and ensuring proper cell function .
Transport and Distribution
Within cells and tissues, this compound is transported and distributed through interactions with specific transporters and binding proteins. These interactions help regulate the localization and accumulation of this compound within the cell, ensuring that it reaches its target sites in the membrane. The distribution of this compound is essential for its function in maintaining membrane stability and protecting cells from environmental stressors .
Subcellular Localization
This compound is primarily localized in the cell membrane, where it exerts its effects on membrane stability and function. It can also be found in other subcellular compartments, such as the cytoplasm and organelles, where it may play additional roles in cellular processes. The subcellular localization of this compound is regulated by targeting signals and post-translational modifications, which direct it to specific compartments within the cell .
Preparation Methods
Synthetic Routes and Reaction Conditions: Hopane and its derivatives are typically synthesized through the cyclization of squalene, a linear triterpene. The enzyme squalene-hopene cyclase catalyzes this reaction, converting squalene into this compound through a cationic cyclization mechanism . The reaction conditions usually involve a buffered solution at a specific pH and temperature to optimize enzyme activity.
Industrial Production Methods: In industrial settings, this compound is often extracted from plants or bacteria. The extraction process involves the use of solvents to isolate this compound from the biological material. Advances in biotechnology have also enabled the production of this compound through microbial fermentation, where genetically engineered bacteria are used to produce this compound in large quantities .
Chemical Reactions Analysis
Types of Reactions: Hopane undergoes various chemical reactions, including oxidation, reduction, and substitution. These reactions are essential for modifying the this compound structure to produce different hopanoids with specific properties.
Common Reagents and Conditions:
Oxidation: Common oxidizing agents such as potassium permanganate or chromium trioxide can be used to oxidize this compound, introducing functional groups like hydroxyl or carbonyl groups.
Reduction: Reducing agents like sodium borohydride or lithium aluminum hydride can reduce this compound derivatives, converting carbonyl groups to hydroxyl groups.
Substitution: Halogenation reactions using reagents like bromine or chlorine can introduce halogen atoms into the this compound structure.
Major Products Formed: The major products formed from these reactions include various hopanoids such as diploptene, diplopterol, and bacteriohopanetetrol .
Scientific Research Applications
Hopane and its derivatives have numerous applications in scientific research:
Comparison with Similar Compounds
Hopane is part of a diverse subclass of triterpenoids known as hopanoids. Similar compounds include:
Diplopterol: Also called hopan-22-ol, it is a hydrated form of diploptene and has similar membrane-stabilizing properties.
Bacteriohopanetetrol: A common extended hopanoid found in bacteria, it plays a role in membrane stability and function.
Uniqueness of this compound: this compound’s unique pentacyclic structure and its ability to form various derivatives make it a versatile compound with wide-ranging applications in different fields. Its stability and functional properties distinguish it from other triterpenoids .
Properties
IUPAC Name |
(3R,3aS,5aR,5bR,7aS,11aS,11bR,13aR,13bS)-5a,5b,8,8,11a,13b-hexamethyl-3-propan-2-yl-1,2,3,3a,4,5,6,7,7a,9,10,11,11b,12,13,13a-hexadecahydrocyclopenta[a]chrysene | |
---|---|---|
Source | PubChem | |
URL | https://pubchem.ncbi.nlm.nih.gov | |
Description | Data deposited in or computed by PubChem | |
InChI |
InChI=1S/C30H52/c1-20(2)21-12-17-27(5)22(21)13-18-29(7)24(27)10-11-25-28(6)16-9-15-26(3,4)23(28)14-19-30(25,29)8/h20-25H,9-19H2,1-8H3/t21-,22+,23+,24-,25-,27+,28+,29-,30-/m1/s1 | |
Source | PubChem | |
URL | https://pubchem.ncbi.nlm.nih.gov | |
Description | Data deposited in or computed by PubChem | |
InChI Key |
ZRLNBWWGLOPJIC-PYQRSULMSA-N | |
Source | PubChem | |
URL | https://pubchem.ncbi.nlm.nih.gov | |
Description | Data deposited in or computed by PubChem | |
Canonical SMILES |
CC(C)C1CCC2(C1CCC3(C2CCC4C3(CCC5C4(CCCC5(C)C)C)C)C)C | |
Source | PubChem | |
URL | https://pubchem.ncbi.nlm.nih.gov | |
Description | Data deposited in or computed by PubChem | |
Isomeric SMILES |
CC(C)[C@H]1CC[C@]2([C@H]1CC[C@@]3([C@@H]2CC[C@H]4[C@]3(CC[C@@H]5[C@@]4(CCCC5(C)C)C)C)C)C | |
Source | PubChem | |
URL | https://pubchem.ncbi.nlm.nih.gov | |
Description | Data deposited in or computed by PubChem | |
Molecular Formula |
C30H52 | |
Source | PubChem | |
URL | https://pubchem.ncbi.nlm.nih.gov | |
Description | Data deposited in or computed by PubChem | |
DSSTOX Substance ID |
DTXSID00904337 | |
Record name | Hopane | |
Source | EPA DSSTox | |
URL | https://comptox.epa.gov/dashboard/DTXSID00904337 | |
Description | DSSTox provides a high quality public chemistry resource for supporting improved predictive toxicology. | |
Molecular Weight |
412.7 g/mol | |
Source | PubChem | |
URL | https://pubchem.ncbi.nlm.nih.gov | |
Description | Data deposited in or computed by PubChem | |
CAS No. |
471-62-5 | |
Record name | Hopane | |
Source | CAS Common Chemistry | |
URL | https://commonchemistry.cas.org/detail?cas_rn=471-62-5 | |
Description | CAS Common Chemistry is an open community resource for accessing chemical information. Nearly 500,000 chemical substances from CAS REGISTRY cover areas of community interest, including common and frequently regulated chemicals, and those relevant to high school and undergraduate chemistry classes. This chemical information, curated by our expert scientists, is provided in alignment with our mission as a division of the American Chemical Society. | |
Explanation | The data from CAS Common Chemistry is provided under a CC-BY-NC 4.0 license, unless otherwise stated. | |
Record name | Hopane | |
Source | ChemIDplus | |
URL | https://pubchem.ncbi.nlm.nih.gov/substance/?source=chemidplus&sourceid=0000471625 | |
Description | ChemIDplus is a free, web search system that provides access to the structure and nomenclature authority files used for the identification of chemical substances cited in National Library of Medicine (NLM) databases, including the TOXNET system. | |
Record name | Hopane | |
Source | EPA DSSTox | |
URL | https://comptox.epa.gov/dashboard/DTXSID00904337 | |
Description | DSSTox provides a high quality public chemistry resource for supporting improved predictive toxicology. | |
Record name | HOPANE | |
Source | FDA Global Substance Registration System (GSRS) | |
URL | https://gsrs.ncats.nih.gov/ginas/app/beta/substances/W2H93796GT | |
Description | The FDA Global Substance Registration System (GSRS) enables the efficient and accurate exchange of information on what substances are in regulated products. Instead of relying on names, which vary across regulatory domains, countries, and regions, the GSRS knowledge base makes it possible for substances to be defined by standardized, scientific descriptions. | |
Explanation | Unless otherwise noted, the contents of the FDA website (www.fda.gov), both text and graphics, are not copyrighted. They are in the public domain and may be republished, reprinted and otherwise used freely by anyone without the need to obtain permission from FDA. Credit to the U.S. Food and Drug Administration as the source is appreciated but not required. | |
Retrosynthesis Analysis
AI-Powered Synthesis Planning: Our tool employs the Template_relevance Pistachio, Template_relevance Bkms_metabolic, Template_relevance Pistachio_ringbreaker, Template_relevance Reaxys, Template_relevance Reaxys_biocatalysis model, leveraging a vast database of chemical reactions to predict feasible synthetic routes.
One-Step Synthesis Focus: Specifically designed for one-step synthesis, it provides concise and direct routes for your target compounds, streamlining the synthesis process.
Accurate Predictions: Utilizing the extensive PISTACHIO, BKMS_METABOLIC, PISTACHIO_RINGBREAKER, REAXYS, REAXYS_BIOCATALYSIS database, our tool offers high-accuracy predictions, reflecting the latest in chemical research and data.
Strategy Settings
Precursor scoring | Relevance Heuristic |
---|---|
Min. plausibility | 0.01 |
Model | Template_relevance |
Template Set | Pistachio/Bkms_metabolic/Pistachio_ringbreaker/Reaxys/Reaxys_biocatalysis |
Top-N result to add to graph | 6 |
Feasible Synthetic Routes
Q1: What is the molecular formula and weight of hopane?
A1: this compound, specifically 17α(H),21β(H)-hopane (C30 this compound), has the molecular formula C30H52 and a molecular weight of 412.7 g/mol.
Q2: Are there different types of hopanes?
A2: Yes, hopanes comprise a large family of pentacyclic triterpenoid compounds. Besides the basic this compound structure, there are numerous variations including homohopanes (C31-C35), norhopanes (missing one or more methyl groups), and rearranged hopanes like diahopanes and neohopanes. [, , , , , ]
Q3: How are hopanes typically characterized?
A3: Gas Chromatography-Mass Spectrometry (GC-MS) is widely used to identify and quantify individual hopanes in complex mixtures like crude oil or sediment extracts. [, , , , , , , , , ] Additional techniques like GC-MS-MS and GC × GC-TOFMS provide enhanced separation and identification, particularly for isomer differentiation. [, ]
Q4: What are the primary sources of hopanes?
A4: Hopanes originate predominantly from bacteria, specifically from the degradation of bacteriohopanepolyols (BHPs), which are components of bacterial cell membranes. [, , , , , , ]
Q5: How are hopanes used as geochemical biomarkers?
A5: Hopanes are resistant to degradation and can persist in sediments for millions of years, making them valuable biomarkers for various applications: [, ]
- Source rock identification: this compound distributions help determine the origin of crude oils and correlate them to their source rocks. [, , , , , , ]
- Depositional environment reconstruction: Specific this compound ratios and the presence of certain hopanoids provide insights into the salinity, redox conditions, and organic matter input of ancient depositional environments. [, , , , , , , , , , , , , ]
- Thermal maturity assessment: The extent of this compound isomerization, such as the ratio of 22S/(22S+22R) homohopanes, serves as a measure of thermal maturity in oils and source rocks. [, , , , , , , ]
- Biodegradation monitoring: While generally resistant, certain hopanes like homohopanes and TAS are susceptible to biodegradation, providing insights into the extent of oil weathering in the environment. [, , , ]
Q6: Can specific hopanoids provide additional information?
A6: Yes, the presence and abundance of specific hopanoids can reveal additional details:
- 2-methylhopanes: Often associated with cyanobacteria and indicate shallow-water carbonate-rich environments. [, , ]
- Gammacerane: Points towards a stratified water column with reducing conditions at depth, often in saline or hypersaline settings. [, , ]
- Oleanane: Suggests input from angiosperms (flowering plants). [, , ]
- 25-norhopanes: Can indicate severe biodegradation in reservoirs or, in some cases, thermal cracking in post-mature source rocks. [, , ]
- 17α(H)-diahopanes: Their high abundance is often linked to specific redox conditions and clay-rich depositional environments. [, , ]
Q7: What are current research areas related to hopanes?
A7: Research continues to explore:
- Hopanoid biosynthesis pathways in bacteria: Understanding the biosynthesis of hopanoid precursors helps interpret their presence in the geological record. [, ]
- Factors influencing hopanoid distributions: This includes the impact of source organisms, environmental factors, diagenesis, and thermal maturity on the final this compound profile observed in sediments and oils. [, , , , , , , , , , , , , ]
- Development of novel this compound-based geochemical proxies: For example, the C32H/C31H ratio is being investigated as a potential redox indicator. []
- Applications of hopanes in environmental forensics: This includes identifying the source of oil spills and assessing the extent of biodegradation in contaminated environments. [, , , ]
Q8: What are potential future applications of this compound research?
A8: Future research may focus on:
Disclaimer and Information on In-Vitro Research Products
Please be aware that all articles and product information presented on BenchChem are intended solely for informational purposes. The products available for purchase on BenchChem are specifically designed for in-vitro studies, which are conducted outside of living organisms. In-vitro studies, derived from the Latin term "in glass," involve experiments performed in controlled laboratory settings using cells or tissues. It is important to note that these products are not categorized as medicines or drugs, and they have not received approval from the FDA for the prevention, treatment, or cure of any medical condition, ailment, or disease. We must emphasize that any form of bodily introduction of these products into humans or animals is strictly prohibited by law. It is essential to adhere to these guidelines to ensure compliance with legal and ethical standards in research and experimentation.