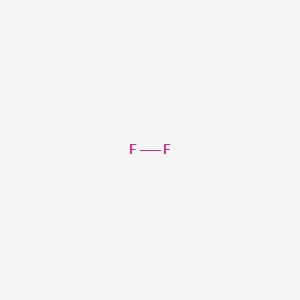
Fluorine
Overview
Description
Fluorine is the ninth element in the periodic table, identified by the atomic symbol “F” and atomic number 9. It is the lightest member of the halogen group and is known for its extreme reactivity and high electronegativity. This compound exists as a pale yellow gas under standard conditions and forms compounds with almost all other elements, except for some noble gases .
Mechanism of Action
Target of Action
This compound, as a small and highly electronegative atom, is often incorporated into biologically active organic compounds to increase their lipophilicity . This property enhances the compound’s ability to interact with various biological targets, including enzymes and receptors. For instance, this compound has been found to inhibit the activity of enzymes such as DNA gyrase and topoisomerase .
Mode of Action
This compound’s mode of action is primarily through its interaction with these targets. The introduction of this compound into a molecule can enhance the binding ability of the molecule to its target proteins . For example, in the case of fluoroquinolones, this compound’s presence inhibits the activity of DNA gyrase and topoisomerase, which are crucial for bacterial DNA replication . This inhibition is fundamentally different from other antibiotics such as penicillin and cephalosporin .
Biochemical Pathways
This compound can affect various biochemical pathways. It is known to inhibit glycolysis, a crucial metabolic pathway, by inhibiting the enzyme enolase . Moreover, fluoride can also compete with Mg2+, an activator of more than 300 enzymes in humans . This competition can disrupt numerous biochemical pathways, leading to various downstream effects.
Pharmacokinetics
This compound’s small size and high electronegativity augment the potency, selectivity, metabolic stability, and pharmacokinetics of drugs . Selective this compound substitution can enhance several pharmacokinetic and physicochemical properties such as metabolic stability and membrane permeation . This often results in increased bioavailability of the drug due to enhanced cell membrane penetration .
Result of Action
The molecular and cellular effects of this compound’s action are diverse. At the molecular level, this compound can cause oxidative stress, organelle damage, and apoptosis in single cells . At the tissue level, the effects of fluoride have been more pronounced in the musculoskeletal systems due to its ability to retain fluoride . Fluoride alters dentinogenesis, thereby affecting tooth enamel formation. In bones, fluoride alters osteogenesis by replacing calcium, resulting in bone deformities .
Action Environment
The action, efficacy, and stability of this compound can be influenced by various environmental factors. For instance, the pH of the environment can affect fluoride absorption, distribution, and excretion . Moreover, the constant increase in this compound compounds emission to the environment leads to a strong fluoride accumulation in living organisms, increasing the risk of the entire ecosystem suffering from fluoride pollution .
Biochemical Analysis
Biochemical Properties
Fluorine plays a crucial role in biochemical reactions due to its high electronegativity and small atomic size. It can form strong hydrogen bonds and interact with various biomolecules. This compound is known to interact with enzymes such as cytochrome P450, where it can act as an inhibitor or modulator of enzyme activity. Additionally, this compound can form stable complexes with proteins, altering their structure and function .
Cellular Effects
This compound’s impact on cellular processes is profound. It can influence cell signaling pathways, gene expression, and cellular metabolism. For instance, this compound can enhance the lipophilicity of drugs, improving their ability to penetrate cell membranes and reach intracellular targets. This property is particularly useful in drug design, where this compound is often incorporated to increase the bioavailability of therapeutic agents .
Molecular Mechanism
At the molecular level, this compound exerts its effects through various mechanisms. It can form covalent bonds with carbon, creating stable fluorinated compounds that are resistant to metabolic degradation. This compound can also interact with biomolecules through hydrogen bonding and van der Waals forces, influencing their conformation and activity. These interactions can lead to enzyme inhibition or activation, as well as changes in gene expression .
Temporal Effects in Laboratory Settings
In laboratory settings, the effects of this compound can change over time. This compound is highly reactive and can degrade or react with other compounds, affecting its stability and long-term effects on cellular function. Studies have shown that prolonged exposure to this compound can lead to oxidative stress and damage to cellular components, highlighting the importance of controlled experimental conditions .
Dosage Effects in Animal Models
The effects of this compound vary with different dosages in animal models. At low doses, this compound can have beneficial effects, such as enhancing bone strength and preventing dental caries. At high doses, this compound can be toxic, causing adverse effects such as skeletal fluorosis and damage to soft tissues. These threshold effects underscore the need for careful dosage regulation in therapeutic applications .
Metabolic Pathways
This compound is involved in various metabolic pathways, interacting with enzymes and cofactors. It can inhibit enzymes involved in glycolysis and the citric acid cycle, affecting metabolic flux and energy production. This compound can also alter metabolite levels, leading to changes in cellular metabolism and function .
Transport and Distribution
Within cells and tissues, this compound is transported and distributed through various mechanisms. It can interact with transporters and binding proteins, influencing its localization and accumulation. This compound’s high lipophilicity allows it to easily cross cell membranes and reach intracellular targets, enhancing its biological activity .
Subcellular Localization
This compound’s subcellular localization is influenced by its chemical properties and interactions with biomolecules. It can be directed to specific compartments or organelles through targeting signals and post-translational modifications. These localization patterns can affect this compound’s activity and function, highlighting the importance of understanding its distribution within cells .
Preparation Methods
Synthetic Routes and Reaction Conditions: Fluorine is primarily produced through the electrolysis of potassium hydrogen fluoride (KHF₂) in anhydrous hydrogen fluoride (HF). This process involves the electrolytic oxidation of HF, which decomposes to form this compound gas at the anode and hydrogen gas at the cathode .
Industrial Production Methods: The industrial production of this compound typically involves the use of a molten mixture of potassium hydrogen fluoride and anhydrous hydrogen fluoride. The electrolysis process is carried out in specialized cells designed to handle the highly reactive and corrosive nature of this compound .
Chemical Reactions Analysis
Types of Reactions: Fluorine undergoes various types of chemical reactions, including:
Oxidation: this compound is a powerful oxidizing agent and can oxidize other elements and compounds.
Reduction: this compound itself is reduced when it gains electrons to form fluoride ions.
Substitution: this compound can replace other halogens in compounds through substitution reactions.
Common Reagents and Conditions: this compound reacts with a wide range of reagents, including metals, non-metals, and organic compounds. Common conditions for these reactions include ambient temperature and pressure, although some reactions may require specific catalysts or solvents .
Major Products: The major products formed from this compound reactions include various fluorides, such as hydrogen fluoride, sodium fluoride, and uranium hexafluoride. These products have significant industrial and scientific applications .
Scientific Research Applications
Fluorine has a wide range of scientific research applications, including:
Chemistry: this compound is used in the synthesis of various organic and inorganic compounds.
Medicine: this compound is incorporated into pharmaceuticals to enhance their stability, bioavailability, and efficacy.
Industry: this compound is used in the production of polymers, refrigerants, and surfactants. .
Comparison with Similar Compounds
- Chlorine (Cl)
- Bromine (Br)
- Iodine (I)
Fluorine’s unique properties and reactivity set it apart from these similar compounds, making it an essential element in various scientific and industrial applications.
Properties
IUPAC Name |
fluoride | |
---|---|---|
Source | PubChem | |
URL | https://pubchem.ncbi.nlm.nih.gov | |
Description | Data deposited in or computed by PubChem | |
InChI |
InChI=1S/FH/h1H/p-1 | |
Source | PubChem | |
URL | https://pubchem.ncbi.nlm.nih.gov | |
Description | Data deposited in or computed by PubChem | |
InChI Key |
KRHYYFGTRYWZRS-UHFFFAOYSA-M | |
Source | PubChem | |
URL | https://pubchem.ncbi.nlm.nih.gov | |
Description | Data deposited in or computed by PubChem | |
Canonical SMILES |
[F-] | |
Source | PubChem | |
URL | https://pubchem.ncbi.nlm.nih.gov | |
Description | Data deposited in or computed by PubChem | |
Molecular Formula |
F- | |
Source | PubChem | |
URL | https://pubchem.ncbi.nlm.nih.gov | |
Description | Data deposited in or computed by PubChem | |
DSSTOX Substance ID |
DTXSID9049617 | |
Record name | Fluoride | |
Source | EPA DSSTox | |
URL | https://comptox.epa.gov/dashboard/DTXSID9049617 | |
Description | DSSTox provides a high quality public chemistry resource for supporting improved predictive toxicology. | |
Molecular Weight |
18.99840316 g/mol | |
Source | PubChem | |
URL | https://pubchem.ncbi.nlm.nih.gov | |
Description | Data deposited in or computed by PubChem | |
Physical Description |
Liquid, Varies depending on specific compound | |
Record name | Fluoride | |
Source | Human Metabolome Database (HMDB) | |
URL | http://www.hmdb.ca/metabolites/HMDB0000662 | |
Description | The Human Metabolome Database (HMDB) is a freely available electronic database containing detailed information about small molecule metabolites found in the human body. | |
Explanation | HMDB is offered to the public as a freely available resource. Use and re-distribution of the data, in whole or in part, for commercial purposes requires explicit permission of the authors and explicit acknowledgment of the source material (HMDB) and the original publication (see the HMDB citing page). We ask that users who download significant portions of the database cite the HMDB paper in any resulting publications. | |
Record name | FLUORIDES (as F) | |
Source | Occupational Safety and Health Administration (OSHA) | |
URL | https://www.osha.gov/chemicaldata/806 | |
Description | The OSHA Occupational Chemical Database contains over 800 entries with information such as physical properties, exposure guidelines, etc. | |
Explanation | Materials created by the federal government are generally part of the public domain and may be used, reproduced and distributed without permission. Therefore, content on this website which is in the public domain may be used without the prior permission of the U.S. Department of Labor (DOL). Warning: Some content - including both images and text - may be the copyrighted property of others and used by the DOL under a license. | |
Solubility |
0.00169 mg/mL at 25 °C | |
Record name | Fluoride | |
Source | Human Metabolome Database (HMDB) | |
URL | http://www.hmdb.ca/metabolites/HMDB0000662 | |
Description | The Human Metabolome Database (HMDB) is a freely available electronic database containing detailed information about small molecule metabolites found in the human body. | |
Explanation | HMDB is offered to the public as a freely available resource. Use and re-distribution of the data, in whole or in part, for commercial purposes requires explicit permission of the authors and explicit acknowledgment of the source material (HMDB) and the original publication (see the HMDB citing page). We ask that users who download significant portions of the database cite the HMDB paper in any resulting publications. | |
CAS No. |
16984-48-8, 7782-41-4 | |
Record name | Fluoride | |
Source | CAS Common Chemistry | |
URL | https://commonchemistry.cas.org/detail?cas_rn=16984-48-8 | |
Description | CAS Common Chemistry is an open community resource for accessing chemical information. Nearly 500,000 chemical substances from CAS REGISTRY cover areas of community interest, including common and frequently regulated chemicals, and those relevant to high school and undergraduate chemistry classes. This chemical information, curated by our expert scientists, is provided in alignment with our mission as a division of the American Chemical Society. | |
Explanation | The data from CAS Common Chemistry is provided under a CC-BY-NC 4.0 license, unless otherwise stated. | |
Record name | Fluoride | |
Source | ChemIDplus | |
URL | https://pubchem.ncbi.nlm.nih.gov/substance/?source=chemidplus&sourceid=0016984488 | |
Description | ChemIDplus is a free, web search system that provides access to the structure and nomenclature authority files used for the identification of chemical substances cited in National Library of Medicine (NLM) databases, including the TOXNET system. | |
Record name | Fluoride ion | |
Source | DrugBank | |
URL | https://www.drugbank.ca/drugs/DB11257 | |
Description | The DrugBank database is a unique bioinformatics and cheminformatics resource that combines detailed drug (i.e. chemical, pharmacological and pharmaceutical) data with comprehensive drug target (i.e. sequence, structure, and pathway) information. | |
Explanation | Creative Common's Attribution-NonCommercial 4.0 International License (http://creativecommons.org/licenses/by-nc/4.0/legalcode) | |
Record name | Fluoride | |
Source | EPA DSSTox | |
URL | https://comptox.epa.gov/dashboard/DTXSID9049617 | |
Description | DSSTox provides a high quality public chemistry resource for supporting improved predictive toxicology. | |
Record name | FLUORIDE ION | |
Source | FDA Global Substance Registration System (GSRS) | |
URL | https://gsrs.ncats.nih.gov/ginas/app/beta/substances/Q80VPU408O | |
Description | The FDA Global Substance Registration System (GSRS) enables the efficient and accurate exchange of information on what substances are in regulated products. Instead of relying on names, which vary across regulatory domains, countries, and regions, the GSRS knowledge base makes it possible for substances to be defined by standardized, scientific descriptions. | |
Explanation | Unless otherwise noted, the contents of the FDA website (www.fda.gov), both text and graphics, are not copyrighted. They are in the public domain and may be republished, reprinted and otherwise used freely by anyone without the need to obtain permission from FDA. Credit to the U.S. Food and Drug Administration as the source is appreciated but not required. | |
Record name | Fluoride | |
Source | Human Metabolome Database (HMDB) | |
URL | http://www.hmdb.ca/metabolites/HMDB0000662 | |
Description | The Human Metabolome Database (HMDB) is a freely available electronic database containing detailed information about small molecule metabolites found in the human body. | |
Explanation | HMDB is offered to the public as a freely available resource. Use and re-distribution of the data, in whole or in part, for commercial purposes requires explicit permission of the authors and explicit acknowledgment of the source material (HMDB) and the original publication (see the HMDB citing page). We ask that users who download significant portions of the database cite the HMDB paper in any resulting publications. | |
Record name | FLUORIDES (as F) | |
Source | Occupational Safety and Health Administration (OSHA) | |
URL | https://www.osha.gov/chemicaldata/806 | |
Description | The OSHA Occupational Chemical Database contains over 800 entries with information such as physical properties, exposure guidelines, etc. | |
Explanation | Materials created by the federal government are generally part of the public domain and may be used, reproduced and distributed without permission. Therefore, content on this website which is in the public domain may be used without the prior permission of the U.S. Department of Labor (DOL). Warning: Some content - including both images and text - may be the copyrighted property of others and used by the DOL under a license. | |
Melting Point |
-219.61 °C | |
Record name | Fluoride | |
Source | Human Metabolome Database (HMDB) | |
URL | http://www.hmdb.ca/metabolites/HMDB0000662 | |
Description | The Human Metabolome Database (HMDB) is a freely available electronic database containing detailed information about small molecule metabolites found in the human body. | |
Explanation | HMDB is offered to the public as a freely available resource. Use and re-distribution of the data, in whole or in part, for commercial purposes requires explicit permission of the authors and explicit acknowledgment of the source material (HMDB) and the original publication (see the HMDB citing page). We ask that users who download significant portions of the database cite the HMDB paper in any resulting publications. | |
Synthesis routes and methods I
Procedure details
Synthesis routes and methods II
Procedure details
Synthesis routes and methods III
Procedure details
Retrosynthesis Analysis
AI-Powered Synthesis Planning: Our tool employs the Template_relevance Pistachio, Template_relevance Bkms_metabolic, Template_relevance Pistachio_ringbreaker, Template_relevance Reaxys, Template_relevance Reaxys_biocatalysis model, leveraging a vast database of chemical reactions to predict feasible synthetic routes.
One-Step Synthesis Focus: Specifically designed for one-step synthesis, it provides concise and direct routes for your target compounds, streamlining the synthesis process.
Accurate Predictions: Utilizing the extensive PISTACHIO, BKMS_METABOLIC, PISTACHIO_RINGBREAKER, REAXYS, REAXYS_BIOCATALYSIS database, our tool offers high-accuracy predictions, reflecting the latest in chemical research and data.
Strategy Settings
Precursor scoring | Relevance Heuristic |
---|---|
Min. plausibility | 0.01 |
Model | Template_relevance |
Template Set | Pistachio/Bkms_metabolic/Pistachio_ringbreaker/Reaxys/Reaxys_biocatalysis |
Top-N result to add to graph | 6 |
Feasible Synthetic Routes
Disclaimer and Information on In-Vitro Research Products
Please be aware that all articles and product information presented on BenchChem are intended solely for informational purposes. The products available for purchase on BenchChem are specifically designed for in-vitro studies, which are conducted outside of living organisms. In-vitro studies, derived from the Latin term "in glass," involve experiments performed in controlled laboratory settings using cells or tissues. It is important to note that these products are not categorized as medicines or drugs, and they have not received approval from the FDA for the prevention, treatment, or cure of any medical condition, ailment, or disease. We must emphasize that any form of bodily introduction of these products into humans or animals is strictly prohibited by law. It is essential to adhere to these guidelines to ensure compliance with legal and ethical standards in research and experimentation.