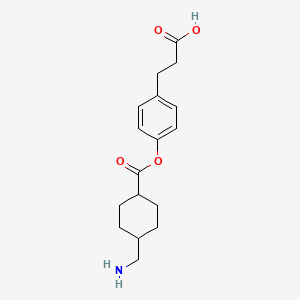
Cetraxate
Overview
Description
Synthesis Analysis
Cetraxate hydrochloride, known for its anti-ulcer properties, has been produced industrially through chemical methods involving the protection of p-hydroxy propionic acid derivatives. A novel enzymatic method for its production involves the use of cellulase enzymes from Aspergillus sp. for the quantitative hydrolysis of this compound benzyl ester hydrochloride into this compound hydrochloride. This enzymatic method offers a selective and efficient route for the synthesis of this compound, showcasing the utility of enzymes in pharmaceutical synthesis (Kuroda, Miyadera, Kaneuchi, & Imura, 1989).
Scientific Research Applications
Gastric Mucosal Blood Flow and Ulcer Healing Cetraxate has been studied for its role in gastric ulcer healing. Adachi et al. (2001) explored the influence of this compound on gastric mucosal blood flow (MBF) during the healing of endoscopic mucosal resection-induced ulcers. They found that this compound might accelerate gastric ulcer healing by augmenting MBF, especially in the context of Helicobacter pylori infection (Adachi et al., 2001).
Impact on Plasma Substances in Healthy Subjects Katagiri et al. (2004) conducted a study on the effects of this compound on human plasma, particularly on calcitonin gene-related peptide (CGRP) and substance P. They observed that this compound administration led to significant increases in plasma CGRP and substance P levels, which might be key mechanisms underlying its gastroprotective action (Katagiri et al., 2004).
This compound vs. Its Metabolites A study by Katagiri et al. (2005) compared the effects of this compound and its major metabolite on human plasma gastrin, somatostatin, CGRP, and substance P. The results indicated that the gastroprotective effect of this compound might not be due to its plasma metabolite, tranexamic acid, but rather due to direct stimulation of the gastric mucosa (Katagiri et al., 2005).
Effects on Stress-Related Hormones Research by Katagiri et al. (2005) also examined the effects of this compound on plasma levels of stress-related hormones such as adrenocorticotropic hormone and cortisol under stress conditions. It was found that this compound had no significant effect on these hormone levels (Katagiri et al., 2005).
Efficacy in Helicobacter Pylori Infection Treatment Wu et al. (2004) investigated the efficacy of this compound-based triple therapy in treating Helicobacter pylori infection. They found that while this compound-based therapy was less effective than pantoprazole-based therapy, it had a lower frequency of adverse effects (Wu et al., 2004).
Development of Sustained-Release Tablets Xie Lan-gui (2006) focused on the development of floating sustained-release tablets for this compound hydrochloride, aiming to achieve a controlled release behavior and sustain drug release for over 6 hours (Xie Lan-gui, 2006).
Comparative Effectiveness in Erosive Gastritis Treatment Seol et al. (2004) compared the effectiveness of DA-9601, a new drug, with this compound in treating erosive gastritis. They found that DA-9601 showed higher cure and improvement rates compared to this compound (Seol et al., 2004).
Role in Gastric Ulcer Healing A Re-evaluation
Higuchi et al. (2007) re-evaluated the effect of gastroprotective drugs like this compound on gastric ulcer healing. They concluded that the superiority of this compound over placebo was established in the context of ulcer healing (Higuchi et al., 2007).
Efficacy on Gastric Mucosal Glycoproteins in Aspirin-induced Gastric Damage Azuumi et al. (2007) studied the efficacy of this compound on mucus glycoproteins in gastric mucosa in aspirin-induced gastric damage. They found that this compound reduced the diminution in mucus glycoproteins caused by aspirin (Azuumi et al., 2007).
Mechanism of Action
Target of Action
Cetraxate, an oral gastrointestinal medication, primarily targets the gastric mucosa . It is believed to indirectly stimulate capsaicin-sensitive afferent nerves, which play a crucial role in maintaining gastric mucosal integrity .
Mode of Action
This compound exerts its effects by increasing mucosal blood flow . This increase in blood flow is thought to be a key mechanism underlying its gastroprotective action . The compound’s interaction with its targets leads to enhanced gastric mucosal defense, thereby reducing the susceptibility of the gastric mucosa to various injuries .
Biochemical Pathways
Substance P is a neuropeptide that acts as a neurotransmitter and neuromodulator, and its increase might indirectly stimulate capsaicin-sensitive afferent nerves .
Result of Action
The primary result of this compound’s action is its cytoprotective effect on the gastric mucosa . It has been shown to be effective in preventing gastric mucosal blood flow decrease in H. pylori-infected patients . This gastroprotective action makes it useful in the treatment of conditions such as acute gastritis or acute exacerbation of chronic gastritis .
Action Environment
The efficacy and stability of this compound can be influenced by various environmental factors. For instance, the presence of H. pylori infection has been shown to affect the drug’s action . Furthermore, factors such as diet, co-administration with other medications, and individual patient characteristics (e.g., age, health status) could potentially influence the drug’s action and efficacy.
Safety and Hazards
properties
IUPAC Name |
3-[4-[4-(aminomethyl)cyclohexanecarbonyl]oxyphenyl]propanoic acid | |
---|---|---|
Source | PubChem | |
URL | https://pubchem.ncbi.nlm.nih.gov | |
Description | Data deposited in or computed by PubChem | |
InChI |
InChI=1S/C17H23NO4/c18-11-13-1-6-14(7-2-13)17(21)22-15-8-3-12(4-9-15)5-10-16(19)20/h3-4,8-9,13-14H,1-2,5-7,10-11,18H2,(H,19,20) | |
Source | PubChem | |
URL | https://pubchem.ncbi.nlm.nih.gov | |
Description | Data deposited in or computed by PubChem | |
InChI Key |
FHRSHSOEWXUORL-UHFFFAOYSA-N | |
Source | PubChem | |
URL | https://pubchem.ncbi.nlm.nih.gov | |
Description | Data deposited in or computed by PubChem | |
Canonical SMILES |
C1CC(CCC1CN)C(=O)OC2=CC=C(C=C2)CCC(=O)O | |
Source | PubChem | |
URL | https://pubchem.ncbi.nlm.nih.gov | |
Description | Data deposited in or computed by PubChem | |
Molecular Formula |
C17H23NO4 | |
Source | PubChem | |
URL | https://pubchem.ncbi.nlm.nih.gov | |
Description | Data deposited in or computed by PubChem | |
DSSTOX Substance ID |
DTXSID2040658, DTXSID60860400 | |
Record name | Cetraxate | |
Source | EPA DSSTox | |
URL | https://comptox.epa.gov/dashboard/DTXSID2040658 | |
Description | DSSTox provides a high quality public chemistry resource for supporting improved predictive toxicology. | |
Record name | 3-(4-{[4-(Aminomethyl)cyclohexane-1-carbonyl]oxy}phenyl)propanoic acid | |
Source | EPA DSSTox | |
URL | https://comptox.epa.gov/dashboard/DTXSID60860400 | |
Description | DSSTox provides a high quality public chemistry resource for supporting improved predictive toxicology. | |
Molecular Weight |
305.4 g/mol | |
Source | PubChem | |
URL | https://pubchem.ncbi.nlm.nih.gov | |
Description | Data deposited in or computed by PubChem | |
CAS RN |
34675-84-8 | |
Record name | Cetraxate [INN] | |
Source | ChemIDplus | |
URL | https://pubchem.ncbi.nlm.nih.gov/substance/?source=chemidplus&sourceid=0034675848 | |
Description | ChemIDplus is a free, web search system that provides access to the structure and nomenclature authority files used for the identification of chemical substances cited in National Library of Medicine (NLM) databases, including the TOXNET system. | |
Record name | Cetraxate | |
Source | EPA DSSTox | |
URL | https://comptox.epa.gov/dashboard/DTXSID2040658 | |
Description | DSSTox provides a high quality public chemistry resource for supporting improved predictive toxicology. | |
Record name | CETRAXATE | |
Source | FDA Global Substance Registration System (GSRS) | |
URL | https://gsrs.ncats.nih.gov/ginas/app/beta/substances/5VPA8CPF0N | |
Description | The FDA Global Substance Registration System (GSRS) enables the efficient and accurate exchange of information on what substances are in regulated products. Instead of relying on names, which vary across regulatory domains, countries, and regions, the GSRS knowledge base makes it possible for substances to be defined by standardized, scientific descriptions. | |
Explanation | Unless otherwise noted, the contents of the FDA website (www.fda.gov), both text and graphics, are not copyrighted. They are in the public domain and may be republished, reprinted and otherwise used freely by anyone without the need to obtain permission from FDA. Credit to the U.S. Food and Drug Administration as the source is appreciated but not required. | |
Retrosynthesis Analysis
AI-Powered Synthesis Planning: Our tool employs the Template_relevance Pistachio, Template_relevance Bkms_metabolic, Template_relevance Pistachio_ringbreaker, Template_relevance Reaxys, Template_relevance Reaxys_biocatalysis model, leveraging a vast database of chemical reactions to predict feasible synthetic routes.
One-Step Synthesis Focus: Specifically designed for one-step synthesis, it provides concise and direct routes for your target compounds, streamlining the synthesis process.
Accurate Predictions: Utilizing the extensive PISTACHIO, BKMS_METABOLIC, PISTACHIO_RINGBREAKER, REAXYS, REAXYS_BIOCATALYSIS database, our tool offers high-accuracy predictions, reflecting the latest in chemical research and data.
Strategy Settings
Precursor scoring | Relevance Heuristic |
---|---|
Min. plausibility | 0.01 |
Model | Template_relevance |
Template Set | Pistachio/Bkms_metabolic/Pistachio_ringbreaker/Reaxys/Reaxys_biocatalysis |
Top-N result to add to graph | 6 |
Feasible Synthetic Routes
Q & A
Q1: How does cetraxate protect the gastric mucosa?
A1: this compound exhibits its gastroprotective action through multiple mechanisms. One key aspect is its ability to increase gastric mucosal blood flow (GMBF). [] This enhanced blood flow supports the natural defenses of the stomach lining, including mucus production, bicarbonate secretion, and cellular regeneration, all crucial for maintaining mucosal integrity. [, ]
Q2: Is the increase in GMBF the sole mechanism behind this compound's gastroprotective effects?
A2: While GMBF enhancement plays a significant role, research suggests that this compound might also directly stimulate the gastric mucosa, contributing to its protective action. [] Additionally, this compound has shown inhibitory effects on certain enzymes like plasmin, casein, and trypsin, which could play a role in its protective mechanism. []
Q3: What is the role of capsaicin-sensitive afferent nerves in this compound's mechanism of action?
A3: this compound has been shown to raise levels of calcitonin gene-related peptide (CGRP) and substance P in human plasma. [] This effect is thought to be linked to the indirect stimulation of capsaicin-sensitive afferent nerves in the stomach, which are known to play a role in gastric mucosal defense. []
Q4: What is the molecular formula and weight of this compound hydrochloride?
A4: The molecular formula of this compound hydrochloride is C20H28N2O5 • HCl, and its molecular weight is 412.9 g/mol.
Q5: How do structural modifications of this compound affect its activity?
A5: While the provided research papers don't delve deeply into specific structural modifications of this compound, they do highlight the structural differences between this compound and its metabolite, tranexamic acid. Interestingly, while tranexamic acid exhibits some anti-ulcer activity, its effect on parameters like plasma CGRP, substance P, gastrin, and somatostatin differs from this compound. [] This suggests that the specific structural features of this compound are essential for its full range of pharmacological activities.
Q6: How is this compound metabolized in the body?
A6: this compound undergoes rapid hydrolysis in the gastrointestinal tract and blood. [] It primarily metabolizes into p-hydroxyphenylpropionic acid (PHPA) and p-hydroxybenzoic acid (PHBA). [] Following oral administration, a significant amount of unchanged this compound is distributed to the gastric wall, while PHPA is found in various organs, excluding the brain. []
Q7: Does tranexamic acid, a metabolite of this compound, contribute to its anti-ulcer activity?
A7: While tranexamic acid itself possesses some anti-ulcer effects, research suggests that this compound's gastroprotective action is primarily attributed to the parent compound rather than its metabolites. [, ] This emphasizes the importance of this compound's unique structure in its pharmacological activity.
Q8: What types of gastric lesions has this compound been shown to be effective against in experimental models?
A8: this compound has demonstrated protective effects against various experimentally induced gastric lesions in animal models, including those induced by:
- HCl/Ethanol []
- Aspirin [, , , , ]
- Water-immersion stress [, , , , ]
- Indomethacin [, , ]
- Pyloric ligation (Shay's ulcer) []
- Phenylbutazone []
- Acetic acid [, , , ]
- Clamping []
- Clamping-cortisone []
- Taurocholate-histamine []
- Taurocholate-serotonin []
- Iodoacetamide []
- Acidified aspirin []
- Acidified ethanol []
Q9: Has this compound been compared to other anti-ulcer drugs in clinical trials?
A9: Yes, this compound has been compared to other anti-ulcer drugs in clinical trials:
- This compound vs. Gefarnate: In a multicenter, double-blind study involving patients with gastric ulcers, this compound demonstrated significantly higher cure rates after 8 and 12 weeks of treatment compared to gefarnate. [] this compound was also found to be superior in improving epigastralgia. []
- This compound vs. Ranitidine: In a double-blind study involving elderly patients with gastric ulcers, ranitidine showed significantly higher ulcer healing rates and earlier pain relief compared to this compound. []
- This compound-based vs. Pantoprazole-based Triple Therapy: A pilot study investigated this compound-based triple therapy (this compound plus clarithromycin and amoxicillin) compared to pantoprazole-based triple therapy for Helicobacter pylori eradication. The pantoprazole-based therapy was significantly more effective, but the this compound-based therapy had fewer side effects. []
Disclaimer and Information on In-Vitro Research Products
Please be aware that all articles and product information presented on BenchChem are intended solely for informational purposes. The products available for purchase on BenchChem are specifically designed for in-vitro studies, which are conducted outside of living organisms. In-vitro studies, derived from the Latin term "in glass," involve experiments performed in controlled laboratory settings using cells or tissues. It is important to note that these products are not categorized as medicines or drugs, and they have not received approval from the FDA for the prevention, treatment, or cure of any medical condition, ailment, or disease. We must emphasize that any form of bodily introduction of these products into humans or animals is strictly prohibited by law. It is essential to adhere to these guidelines to ensure compliance with legal and ethical standards in research and experimentation.