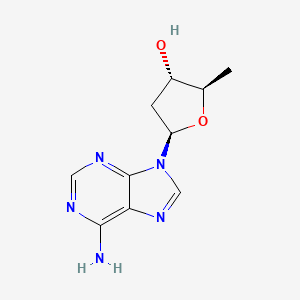
2',5'-Dideoxyadenosine
Overview
Description
2’,5’-Dideoxyadenosine is a nucleoside analog that acts as a potent and non-competitive inhibitor of adenylyl cyclase . This compound is known for its ability to inhibit the production of cyclic adenosine monophosphate (cAMP), a crucial second messenger in various biological processes . It has significant applications in scientific research, particularly in the fields of biochemistry and pharmacology .
Mechanism of Action
Target of Action
The primary target of 2’,5’-Dideoxyadenosine is adenylyl cyclase, an enzyme involved in the conversion of ATP to cyclic AMP (cAMP) and pyrophosphate . This enzyme plays a crucial role in intracellular signal transduction .
Mode of Action
2’,5’-Dideoxyadenosine acts as a potent and non-competitive inhibitor of adenylyl cyclase . It binds to the P-site of the enzyme, effectively inhibiting its activity with an IC50 of 3 µM . As a nucleoside analog, it exerts a potent antiadrenergic action in the heart .
Biochemical Pathways
By inhibiting adenylyl cyclase, 2’,5’-Dideoxyadenosine reduces the production of cAMP . This can block the phosphorylation of GluA1 at the Ser845 site induced by carbachol (CCh) . It also blocks CCh-induced increases in Akt phosphorylation .
Pharmacokinetics
It’s known that the compound is cell-permeable , which suggests it can readily cross cell membranes to exert its effects.
Result of Action
The inhibition of adenylyl cyclase by 2’,5’-Dideoxyadenosine leads to a decrease in cAMP production . This can have various downstream effects, including the modulation of heart function due to its antiadrenergic action .
Action Environment
It’s worth noting that the inhibitory effect of 2’,5’-dideoxyadenosine on adenylyl cyclase can vary widely among tissues .
Biochemical Analysis
Biochemical Properties
2’,5’-Dideoxyadenosine plays a crucial role in biochemical reactions, primarily as a non-competitive inhibitor of adenylate cyclase. This enzyme catalyzes the conversion of adenosine triphosphate (ATP) to cyclic adenosine monophosphate (cAMP), a key second messenger in cellular signaling pathways . By binding to the adenosine P1 binding site, 2’,5’-Dideoxyadenosine effectively inhibits adenylate cyclase activity, leading to a decrease in cAMP levels . This inhibition can vary widely among different tissues, with IC₅₀ values ranging from 3 µM in rat brain membranes to 45 µM in purified bovine brain enzyme .
Cellular Effects
2’,5’-Dideoxyadenosine exerts significant effects on various cell types and cellular processes. By inhibiting adenylate cyclase, it reduces cAMP levels, which in turn affects multiple downstream signaling pathways. This reduction in cAMP can lead to decreased activation of cAMP-dependent protein kinase (PKA), altered ion channel activity, and changes in gene expression . Additionally, 2’,5’-Dideoxyadenosine has been shown to block the positive inotropic and chronotropic effects of β-adrenergic agents, further influencing cellular metabolism and function .
Molecular Mechanism
At the molecular level, 2’,5’-Dideoxyadenosine exerts its effects by binding non-competitively to the P-site of adenylate cyclase. This binding inhibits the enzyme’s activity, preventing the conversion of ATP to cAMP . The compound’s inhibitory effect is not reversible and does not compete with ATP, making it a potent and specific inhibitor of adenylate cyclase . This mechanism of action is crucial for understanding how 2’,5’-Dideoxyadenosine modulates cellular signaling and metabolic pathways.
Temporal Effects in Laboratory Settings
In laboratory settings, the effects of 2’,5’-Dideoxyadenosine can change over time. The compound is generally stable under standard storage conditions, but its inhibitory effects on adenylate cyclase can vary depending on the experimental setup and duration . Long-term studies have shown that 2’,5’-Dideoxyadenosine can maintain its inhibitory activity over extended periods, although the degree of inhibition may decrease with prolonged exposure . Additionally, the compound’s stability and degradation rates can influence its effectiveness in in vitro and in vivo studies .
Dosage Effects in Animal Models
The effects of 2’,5’-Dideoxyadenosine in animal models vary with different dosages. At lower doses, the compound effectively inhibits adenylate cyclase without causing significant adverse effects . At higher doses, 2’,5’-Dideoxyadenosine can exhibit toxic effects, including disruptions in cellular metabolism and potential cytotoxicity . These dosage-dependent effects highlight the importance of careful dose optimization in experimental studies to balance efficacy and safety.
Metabolic Pathways
2’,5’-Dideoxyadenosine is involved in several metabolic pathways, primarily through its interaction with adenylate cyclase . The compound’s inhibition of adenylate cyclase affects the levels of cAMP, which in turn influences various metabolic processes . Additionally, 2’,5’-Dideoxyadenosine can be metabolized by enzymes such as adenosine kinase and deoxycytidine kinase, further impacting its activity and stability within cells .
Transport and Distribution
Within cells and tissues, 2’,5’-Dideoxyadenosine is transported and distributed through various mechanisms. The compound is cell-permeable, allowing it to cross cell membranes and reach its target sites . Once inside the cell, 2’,5’-Dideoxyadenosine can interact with specific transporters and binding proteins that facilitate its distribution and localization . These interactions are crucial for the compound’s effectiveness in inhibiting adenylate cyclase and modulating cellular functions.
Subcellular Localization
The subcellular localization of 2’,5’-Dideoxyadenosine is essential for its activity and function. The compound primarily localizes to the cytoplasm, where it interacts with adenylate cyclase and other target proteins . Additionally, 2’,5’-Dideoxyadenosine may be directed to specific cellular compartments or organelles through targeting signals or post-translational modifications
Preparation Methods
Synthetic Routes and Reaction Conditions: The synthesis of 2’,5’-dideoxyadenosine typically involves the reduction of nucleosides. One common method includes the reduction of a nucleoside with hydrogen in the presence of a palladium catalyst and an aqueous solvent mixture of acetonitrile or ethyl acetate and water . The solvent mixture contains a base such as sodium hydroxide/sodium acetate or sodium carbonate/sodium acetate, with a pH of 9-11 .
Industrial Production Methods: While specific industrial production methods for 2’,5’-dideoxyadenosine are not extensively documented, the general approach involves large-scale chemical synthesis using the aforementioned reduction techniques. The process is optimized for yield and purity to meet industrial standards .
Chemical Reactions Analysis
Types of Reactions: 2’,5’-Dideoxyadenosine undergoes various chemical reactions, including:
Oxidation: This reaction can modify the nucleoside structure, potentially altering its biological activity.
Reduction: As part of its synthesis, reduction reactions are crucial for removing specific functional groups.
Substitution: This reaction involves replacing one functional group with another, which can be used to create derivatives of 2’,5’-dideoxyadenosine.
Common Reagents and Conditions:
Oxidation: Common oxidizing agents include potassium permanganate and hydrogen peroxide.
Reduction: Hydrogen gas in the presence of a palladium catalyst is frequently used.
Substitution: Various halogenating agents and nucleophiles are employed depending on the desired substitution.
Major Products: The primary product of these reactions is 2’,5’-dideoxyadenosine itself, along with its various derivatives, which may have different biological activities and applications .
Scientific Research Applications
2’,5’-Dideoxyadenosine has a wide range of applications in scientific research:
Biochemistry: It is used to study the inhibition of adenylyl cyclase and the resulting effects on cAMP production.
Pharmacology: The compound is employed to investigate its antiadrenergic effects in the heart and its potential therapeutic applications.
Cell Biology: It is used to study cell differentiation and growth inhibition, particularly in cancer research.
Comparison with Similar Compounds
2’,3’-Dideoxyadenosine: Another nucleoside analog with similar inhibitory effects on adenylyl cyclase.
Didanosine: A nucleoside analog used as an antiviral agent, particularly in the treatment of HIV.
2-Chloro-2’-deoxyadenosine: A compound with similar structural features but different biological activities.
Uniqueness: 2’,5’-Dideoxyadenosine is unique due to its specific inhibition of adenylyl cyclase and its potent antiadrenergic effects. Its ability to permeate cell membranes and exert its effects intracellularly distinguishes it from other similar compounds .
Properties
IUPAC Name |
5-(6-aminopurin-9-yl)-2-methyloxolan-3-ol | |
---|---|---|
Details | Computed by Lexichem TK 2.7.0 (PubChem release 2021.05.07) | |
Source | PubChem | |
URL | https://pubchem.ncbi.nlm.nih.gov | |
Description | Data deposited in or computed by PubChem | |
InChI |
InChI=1S/C10H13N5O2/c1-5-6(16)2-7(17-5)15-4-14-8-9(11)12-3-13-10(8)15/h3-7,16H,2H2,1H3,(H2,11,12,13) | |
Details | Computed by InChI 1.0.6 (PubChem release 2021.05.07) | |
Source | PubChem | |
URL | https://pubchem.ncbi.nlm.nih.gov | |
Description | Data deposited in or computed by PubChem | |
InChI Key |
FFHPXOJTVQDVMO-UHFFFAOYSA-N | |
Details | Computed by InChI 1.0.6 (PubChem release 2021.05.07) | |
Source | PubChem | |
URL | https://pubchem.ncbi.nlm.nih.gov | |
Description | Data deposited in or computed by PubChem | |
Canonical SMILES |
CC1C(CC(O1)N2C=NC3=C(N=CN=C32)N)O | |
Details | Computed by OEChem 2.3.0 (PubChem release 2021.05.07) | |
Source | PubChem | |
URL | https://pubchem.ncbi.nlm.nih.gov | |
Description | Data deposited in or computed by PubChem | |
Molecular Formula |
C10H13N5O2 | |
Details | Computed by PubChem 2.1 (PubChem release 2021.05.07) | |
Source | PubChem | |
URL | https://pubchem.ncbi.nlm.nih.gov | |
Description | Data deposited in or computed by PubChem | |
DSSTOX Substance ID |
DTXSID90927095 | |
Record name | 9-(2,5-Dideoxypentofuranosyl)-9H-purin-6-amine | |
Source | EPA DSSTox | |
URL | https://comptox.epa.gov/dashboard/DTXSID90927095 | |
Description | DSSTox provides a high quality public chemistry resource for supporting improved predictive toxicology. | |
Molecular Weight |
235.24 g/mol | |
Details | Computed by PubChem 2.1 (PubChem release 2021.05.07) | |
Source | PubChem | |
URL | https://pubchem.ncbi.nlm.nih.gov | |
Description | Data deposited in or computed by PubChem | |
CAS No. |
6698-26-6, 13116-42-2 | |
Record name | NSC95943 | |
Source | DTP/NCI | |
URL | https://dtp.cancer.gov/dtpstandard/servlet/dwindex?searchtype=NSC&outputformat=html&searchlist=95943 | |
Description | The NCI Development Therapeutics Program (DTP) provides services and resources to the academic and private-sector research communities worldwide to facilitate the discovery and development of new cancer therapeutic agents. | |
Explanation | Unless otherwise indicated, all text within NCI products is free of copyright and may be reused without our permission. Credit the National Cancer Institute as the source. | |
Record name | NSC95107 | |
Source | DTP/NCI | |
URL | https://dtp.cancer.gov/dtpstandard/servlet/dwindex?searchtype=NSC&outputformat=html&searchlist=95107 | |
Description | The NCI Development Therapeutics Program (DTP) provides services and resources to the academic and private-sector research communities worldwide to facilitate the discovery and development of new cancer therapeutic agents. | |
Explanation | Unless otherwise indicated, all text within NCI products is free of copyright and may be reused without our permission. Credit the National Cancer Institute as the source. | |
Record name | 9-(2,5-Dideoxypentofuranosyl)-9H-purin-6-amine | |
Source | EPA DSSTox | |
URL | https://comptox.epa.gov/dashboard/DTXSID90927095 | |
Description | DSSTox provides a high quality public chemistry resource for supporting improved predictive toxicology. | |
Retrosynthesis Analysis
AI-Powered Synthesis Planning: Our tool employs the Template_relevance Pistachio, Template_relevance Bkms_metabolic, Template_relevance Pistachio_ringbreaker, Template_relevance Reaxys, Template_relevance Reaxys_biocatalysis model, leveraging a vast database of chemical reactions to predict feasible synthetic routes.
One-Step Synthesis Focus: Specifically designed for one-step synthesis, it provides concise and direct routes for your target compounds, streamlining the synthesis process.
Accurate Predictions: Utilizing the extensive PISTACHIO, BKMS_METABOLIC, PISTACHIO_RINGBREAKER, REAXYS, REAXYS_BIOCATALYSIS database, our tool offers high-accuracy predictions, reflecting the latest in chemical research and data.
Strategy Settings
Precursor scoring | Relevance Heuristic |
---|---|
Min. plausibility | 0.01 |
Model | Template_relevance |
Template Set | Pistachio/Bkms_metabolic/Pistachio_ringbreaker/Reaxys/Reaxys_biocatalysis |
Top-N result to add to graph | 6 |
Feasible Synthetic Routes
Disclaimer and Information on In-Vitro Research Products
Please be aware that all articles and product information presented on BenchChem are intended solely for informational purposes. The products available for purchase on BenchChem are specifically designed for in-vitro studies, which are conducted outside of living organisms. In-vitro studies, derived from the Latin term "in glass," involve experiments performed in controlled laboratory settings using cells or tissues. It is important to note that these products are not categorized as medicines or drugs, and they have not received approval from the FDA for the prevention, treatment, or cure of any medical condition, ailment, or disease. We must emphasize that any form of bodily introduction of these products into humans or animals is strictly prohibited by law. It is essential to adhere to these guidelines to ensure compliance with legal and ethical standards in research and experimentation.