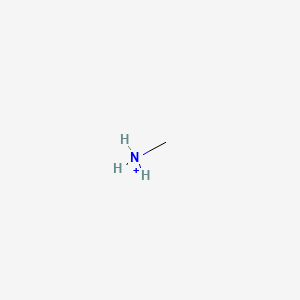
Methylammonium
- Click on QUICK INQUIRY to receive a quote from our team of experts.
- With the quality product at a COMPETITIVE price, you can focus more on your research.
Overview
Description
Methylammonium is the conjugate acid of methylamine; major species at pH 7.3. It has a role as a human metabolite. It is a conjugate acid of a methylamine.
Scientific Research Applications
Solar Energy Applications
Methylammonium Lead Halide Perovskites
This compound lead halides, such as this compound lead iodide (MAPbI₃), have emerged as promising materials for solar cell technology due to their unique properties:
- High Absorption Coefficients : These materials exhibit high absorption coefficients, making them efficient at converting sunlight into electricity.
- Variable Band Gaps : The tunability of band gaps allows for optimization in different solar cell configurations.
- Solution-Processable Synthesis : This technique enables low-cost production methods, enhancing scalability for commercial applications.
Performance Metrics
Perovskite Type | Thickness (nm) | Power Conversion Efficiency (%) |
---|---|---|
MAPbI₃ | 250 | 13.66 |
MAPbBr₃ | 500 | 6.87 |
MAPbCl₃ | 500 | 4.98 |
The study indicates that MAPbI₃ is the most effective candidate for solar cells due to its superior efficiency and structural properties .
Optoelectronic Devices
Photodetectors and Light Emitting Diodes
This compound-based perovskites are also utilized in photodetectors and light-emitting diodes (LEDs). Their ability to emit light efficiently and respond to light makes them suitable for these applications.
- Photodetectors : The use of this compound lead bromide (MAPbBr₃) has shown promise in detecting light with high sensitivity and rapid response times.
- Light Emitting Diodes : this compound perovskites can be engineered to emit specific wavelengths, making them versatile for various lighting applications.
Case Study: this compound Lead Bromide
Research demonstrates that this compound lead bromide can serve as a photoactive layer in optoelectronic devices, exhibiting favorable electronic properties that enhance device performance .
Health Sciences
Toxicological Studies
While this compound compounds show great potential in energy applications, their health impacts are under investigation. Studies have focused on the toxicity of this compound lead iodide on human cells:
- Cellular Effects : Exposure to this compound lead iodide resulted in morphological changes in lung adenocarcinoma cells, including increased cell size and mitochondrial activity .
- Lead Toxicity : The presence of lead raises concerns about environmental and health safety, prompting research into non-toxic alternatives .
Properties
Molecular Formula |
CH6N+ |
---|---|
Molecular Weight |
32.065 g/mol |
IUPAC Name |
methylazanium |
InChI |
InChI=1S/CH5N/c1-2/h2H2,1H3/p+1 |
InChI Key |
BAVYZALUXZFZLV-UHFFFAOYSA-O |
SMILES |
C[NH3+] |
Canonical SMILES |
C[NH3+] |
Synonyms |
aminomethane methylamine methylamine bisulfite methylamine hydride methylamine hydrobromide methylamine hydrochloride methylamine hydrochloride, 14C-labeled methylamine hydrofluoride methylamine hydrogen cyanide methylamine hydroiodide methylamine ion (1-) methylamine nitrate methylamine perchlorate methylamine sulfate (1:1) methylamine sulfate (2:1) methylamine, 13C-labeled methylamine, 14C-labeled methylamine, 15N-labeled methylamine, cesium salt methylamine, monopotassium salt methylamine, monosodium salt methylammonium methylammonium ion monomethylamine monomethylammonium ion |
Origin of Product |
United States |
Retrosynthesis Analysis
AI-Powered Synthesis Planning: Our tool employs the Template_relevance Pistachio, Template_relevance Bkms_metabolic, Template_relevance Pistachio_ringbreaker, Template_relevance Reaxys, Template_relevance Reaxys_biocatalysis model, leveraging a vast database of chemical reactions to predict feasible synthetic routes.
One-Step Synthesis Focus: Specifically designed for one-step synthesis, it provides concise and direct routes for your target compounds, streamlining the synthesis process.
Accurate Predictions: Utilizing the extensive PISTACHIO, BKMS_METABOLIC, PISTACHIO_RINGBREAKER, REAXYS, REAXYS_BIOCATALYSIS database, our tool offers high-accuracy predictions, reflecting the latest in chemical research and data.
Strategy Settings
Precursor scoring | Relevance Heuristic |
---|---|
Min. plausibility | 0.01 |
Model | Template_relevance |
Template Set | Pistachio/Bkms_metabolic/Pistachio_ringbreaker/Reaxys/Reaxys_biocatalysis |
Top-N result to add to graph | 6 |
Feasible Synthetic Routes
Disclaimer and Information on In-Vitro Research Products
Please be aware that all articles and product information presented on BenchChem are intended solely for informational purposes. The products available for purchase on BenchChem are specifically designed for in-vitro studies, which are conducted outside of living organisms. In-vitro studies, derived from the Latin term "in glass," involve experiments performed in controlled laboratory settings using cells or tissues. It is important to note that these products are not categorized as medicines or drugs, and they have not received approval from the FDA for the prevention, treatment, or cure of any medical condition, ailment, or disease. We must emphasize that any form of bodily introduction of these products into humans or animals is strictly prohibited by law. It is essential to adhere to these guidelines to ensure compliance with legal and ethical standards in research and experimentation.