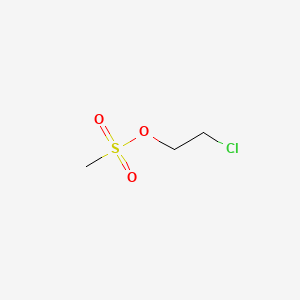
2-Chloroethyl methanesulfonate
Overview
Description
2-Chloroethyl methanesulfonate, also known as this compound, is a useful research compound. Its molecular formula is C3H7ClO3S and its molecular weight is 158.60 g/mol. The purity is usually 95%.
The exact mass of the compound this compound is unknown and the complexity rating of the compound is unknown. The compound has been submitted to the National Cancer Institute (NCI) for testing and evaluation and the Cancer Chemotherapy National Service Center (NSC) number is 18016. The United Nations designated GHS hazard class pictogram is Irritant;Health Hazard, and the GHS signal word is WarningThe storage condition is unknown. Please store according to label instructions upon receipt of goods.
BenchChem offers high-quality this compound suitable for many research applications. Different packaging options are available to accommodate customers' requirements. Please inquire for more information about this compound including the price, delivery time, and more detailed information at info@benchchem.com.
Mechanism of Action
Target of Action
2-Chloroethyl methanesulfonate (2ClEMS) is primarily an alkylating agent . Its primary targets are nucleophilic sites in biomolecules, particularly those found in DNA.
Mode of Action
The mode of action of 2ClEMS involves its alkylating properties. The electrophilic carbon in the compound attacks nucleophilic sites in biomolecules, forming a covalent bond and introducing an ethyl group. This modification can alter the molecule’s function, stability, or interaction with other molecules.
Biochemical Pathways
The primary biochemical pathway affected by 2ClEMS is DNA alkylation . This process involves the addition of an alkyl group to the DNA molecule, which can lead to mutations and changes in gene expression . The compound has been shown to induce alcohol dehydrogenase (Adh) null germline mutation frequency in treated Drosophila melanogaster second instar larval gonia .
Pharmacokinetics
It is known that the compound is highly soluble in water , suggesting that it may be readily absorbed and distributed in the body. More research is needed to fully understand the ADME properties of 2ClEMS and their impact on its bioavailability.
Result of Action
The primary result of 2ClEMS action is the induction of mutations in DNA . This can lead to changes in gene expression and potentially contribute to the development of diseases such as cancer . The compound has been shown to induce a significant increase in mutation frequency in treated Drosophila melanogaster second instar larval gonia .
Action Environment
The action of 2ClEMS can be influenced by various environmental factors. For instance, the compound’s solubility in water suggests that it may be more active in aqueous environments . Additionally, the compound’s reactivity may be influenced by pH, temperature, and the presence of other chemicals.
Biochemical Analysis
Biochemical Properties
2-Chloroethyl methanesulfonate acts as an alkylating agent, meaning it can add alkyl groups to DNA, proteins, and other biomolecules. This compound interacts with enzymes, proteins, and nucleic acids, leading to modifications that can alter their function. For example, it can form covalent bonds with the guanine bases in DNA, leading to the formation of DNA cross-links. These cross-links can interfere with DNA replication and transcription, ultimately affecting cell division and function .
Cellular Effects
The effects of this compound on cells are profound. It can induce DNA damage, leading to cell cycle arrest and apoptosis (programmed cell death). This compound has been shown to produce DNA interstrand cross-links, DNA-protein cross-links, and DNA strand breaks . These effects can disrupt cell signaling pathways, alter gene expression, and impair cellular metabolism. In cancer cells, these disruptions can lead to cell death, making this compound a potential chemotherapeutic agent .
Molecular Mechanism
At the molecular level, this compound exerts its effects by alkylating DNA and proteins. The compound’s chloroethyl group reacts with the nucleophilic sites in DNA, particularly the N7 position of guanine, forming covalent bonds. This alkylation can result in the formation of DNA cross-links, which prevent the separation of DNA strands during replication and transcription . Additionally, this compound can inhibit or activate enzymes by modifying their active sites, further affecting cellular processes .
Temporal Effects in Laboratory Settings
In laboratory settings, the effects of this compound can change over time. The compound is relatively stable, but it can degrade under certain conditions, such as exposure to light or heat . Over time, the DNA damage induced by this compound can be repaired by cellular mechanisms, although some damage may persist and lead to long-term effects on cellular function . In in vitro studies, the compound has been shown to produce immediate DNA damage, followed by delayed repair processes .
Dosage Effects in Animal Models
The effects of this compound vary with different dosages in animal models. At low doses, the compound can induce DNA damage and cell cycle arrest without causing significant toxicity . At higher doses, it can lead to severe toxicity, including bone marrow suppression, gastrointestinal toxicity, and neurotoxicity . The therapeutic window for this compound is narrow, and careful dosing is required to balance its antitumor effects with its potential toxicities .
Metabolic Pathways
This compound is metabolized in the body through various pathways. It can be hydrolyzed to form methanesulfonic acid and 2-chloroethanol, which can further undergo oxidation and conjugation reactions . Enzymes such as cytochrome P450s and glutathione S-transferases may be involved in the metabolism of this compound, affecting its bioavailability and toxicity . The compound’s metabolites can also interact with cellular components, contributing to its overall biological effects .
Transport and Distribution
Within cells and tissues, this compound is transported and distributed through passive diffusion and active transport mechanisms . It can interact with transporters and binding proteins, influencing its localization and accumulation in specific cellular compartments . The compound’s lipophilic nature allows it to cross cell membranes easily, facilitating its distribution throughout the body .
Subcellular Localization
This compound can localize to various subcellular compartments, including the nucleus, cytoplasm, and mitochondria . Its localization is influenced by targeting signals and post-translational modifications that direct it to specific organelles . In the nucleus, this compound can interact with DNA and nuclear proteins, leading to the formation of DNA adducts and cross-links . In the cytoplasm, it can modify cytosolic proteins, affecting their function and stability .
Properties
IUPAC Name |
2-chloroethyl methanesulfonate | |
---|---|---|
Source | PubChem | |
URL | https://pubchem.ncbi.nlm.nih.gov | |
Description | Data deposited in or computed by PubChem | |
InChI |
InChI=1S/C3H7ClO3S/c1-8(5,6)7-3-2-4/h2-3H2,1H3 | |
Source | PubChem | |
URL | https://pubchem.ncbi.nlm.nih.gov | |
Description | Data deposited in or computed by PubChem | |
InChI Key |
FMMYTRQXHORTCU-UHFFFAOYSA-N | |
Source | PubChem | |
URL | https://pubchem.ncbi.nlm.nih.gov | |
Description | Data deposited in or computed by PubChem | |
Canonical SMILES |
CS(=O)(=O)OCCCl | |
Source | PubChem | |
URL | https://pubchem.ncbi.nlm.nih.gov | |
Description | Data deposited in or computed by PubChem | |
Molecular Formula |
C3H7ClO3S | |
Source | PubChem | |
URL | https://pubchem.ncbi.nlm.nih.gov | |
Description | Data deposited in or computed by PubChem | |
DSSTOX Substance ID |
DTXSID20189220 | |
Record name | 2-Chloroethyl methanesulfonate | |
Source | EPA DSSTox | |
URL | https://comptox.epa.gov/dashboard/DTXSID20189220 | |
Description | DSSTox provides a high quality public chemistry resource for supporting improved predictive toxicology. | |
Molecular Weight |
158.60 g/mol | |
Source | PubChem | |
URL | https://pubchem.ncbi.nlm.nih.gov | |
Description | Data deposited in or computed by PubChem | |
CAS No. |
3570-58-9 | |
Record name | Ethanol, 2-chloro-, 1-methanesulfonate | |
Source | CAS Common Chemistry | |
URL | https://commonchemistry.cas.org/detail?cas_rn=3570-58-9 | |
Description | CAS Common Chemistry is an open community resource for accessing chemical information. Nearly 500,000 chemical substances from CAS REGISTRY cover areas of community interest, including common and frequently regulated chemicals, and those relevant to high school and undergraduate chemistry classes. This chemical information, curated by our expert scientists, is provided in alignment with our mission as a division of the American Chemical Society. | |
Explanation | The data from CAS Common Chemistry is provided under a CC-BY-NC 4.0 license, unless otherwise stated. | |
Record name | 2-Chloroethyl methanesulfonate | |
Source | ChemIDplus | |
URL | https://pubchem.ncbi.nlm.nih.gov/substance/?source=chemidplus&sourceid=0003570589 | |
Description | ChemIDplus is a free, web search system that provides access to the structure and nomenclature authority files used for the identification of chemical substances cited in National Library of Medicine (NLM) databases, including the TOXNET system. | |
Record name | 2-CHLOROETHYL METHANESULFONATE | |
Source | DTP/NCI | |
URL | https://dtp.cancer.gov/dtpstandard/servlet/dwindex?searchtype=NSC&outputformat=html&searchlist=18016 | |
Description | The NCI Development Therapeutics Program (DTP) provides services and resources to the academic and private-sector research communities worldwide to facilitate the discovery and development of new cancer therapeutic agents. | |
Explanation | Unless otherwise indicated, all text within NCI products is free of copyright and may be reused without our permission. Credit the National Cancer Institute as the source. | |
Record name | 2-Chloroethyl methanesulfonate | |
Source | EPA DSSTox | |
URL | https://comptox.epa.gov/dashboard/DTXSID20189220 | |
Description | DSSTox provides a high quality public chemistry resource for supporting improved predictive toxicology. | |
Record name | 2-chloroethyl methanesulphonate | |
Source | European Chemicals Agency (ECHA) | |
URL | https://echa.europa.eu/substance-information/-/substanceinfo/100.020.611 | |
Description | The European Chemicals Agency (ECHA) is an agency of the European Union which is the driving force among regulatory authorities in implementing the EU's groundbreaking chemicals legislation for the benefit of human health and the environment as well as for innovation and competitiveness. | |
Explanation | Use of the information, documents and data from the ECHA website is subject to the terms and conditions of this Legal Notice, and subject to other binding limitations provided for under applicable law, the information, documents and data made available on the ECHA website may be reproduced, distributed and/or used, totally or in part, for non-commercial purposes provided that ECHA is acknowledged as the source: "Source: European Chemicals Agency, http://echa.europa.eu/". Such acknowledgement must be included in each copy of the material. ECHA permits and encourages organisations and individuals to create links to the ECHA website under the following cumulative conditions: Links can only be made to webpages that provide a link to the Legal Notice page. | |
Record name | 2-CHLOROETHYL METHANESULFONATE | |
Source | FDA Global Substance Registration System (GSRS) | |
URL | https://gsrs.ncats.nih.gov/ginas/app/beta/substances/895H18Q125 | |
Description | The FDA Global Substance Registration System (GSRS) enables the efficient and accurate exchange of information on what substances are in regulated products. Instead of relying on names, which vary across regulatory domains, countries, and regions, the GSRS knowledge base makes it possible for substances to be defined by standardized, scientific descriptions. | |
Explanation | Unless otherwise noted, the contents of the FDA website (www.fda.gov), both text and graphics, are not copyrighted. They are in the public domain and may be republished, reprinted and otherwise used freely by anyone without the need to obtain permission from FDA. Credit to the U.S. Food and Drug Administration as the source is appreciated but not required. | |
Retrosynthesis Analysis
AI-Powered Synthesis Planning: Our tool employs the Template_relevance Pistachio, Template_relevance Bkms_metabolic, Template_relevance Pistachio_ringbreaker, Template_relevance Reaxys, Template_relevance Reaxys_biocatalysis model, leveraging a vast database of chemical reactions to predict feasible synthetic routes.
One-Step Synthesis Focus: Specifically designed for one-step synthesis, it provides concise and direct routes for your target compounds, streamlining the synthesis process.
Accurate Predictions: Utilizing the extensive PISTACHIO, BKMS_METABOLIC, PISTACHIO_RINGBREAKER, REAXYS, REAXYS_BIOCATALYSIS database, our tool offers high-accuracy predictions, reflecting the latest in chemical research and data.
Strategy Settings
Precursor scoring | Relevance Heuristic |
---|---|
Min. plausibility | 0.01 |
Model | Template_relevance |
Template Set | Pistachio/Bkms_metabolic/Pistachio_ringbreaker/Reaxys/Reaxys_biocatalysis |
Top-N result to add to graph | 6 |
Feasible Synthetic Routes
Disclaimer and Information on In-Vitro Research Products
Please be aware that all articles and product information presented on BenchChem are intended solely for informational purposes. The products available for purchase on BenchChem are specifically designed for in-vitro studies, which are conducted outside of living organisms. In-vitro studies, derived from the Latin term "in glass," involve experiments performed in controlled laboratory settings using cells or tissues. It is important to note that these products are not categorized as medicines or drugs, and they have not received approval from the FDA for the prevention, treatment, or cure of any medical condition, ailment, or disease. We must emphasize that any form of bodily introduction of these products into humans or animals is strictly prohibited by law. It is essential to adhere to these guidelines to ensure compliance with legal and ethical standards in research and experimentation.