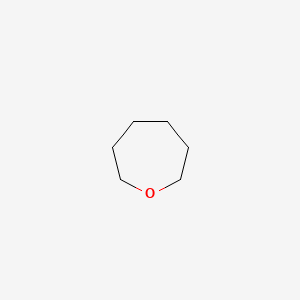
Oxepane
Overview
Description
Oxepane, a seven-membered heterocyclic compound, features prominently in various chemical syntheses and applications. Its unique structural properties make it a subject of considerable interest in organic chemistry.
Synthesis Analysis
- The synthesis of oxepanes involves modern cyclization procedures such as ring-closing metathesis and transformations of carbo- and heterocyclic compounds, highlighting the versatility and complexity of creating these compounds (Belen’kii, 2008).
- Organocatalytic oxa-conjugate addition reactions have been employed to overcome the synthetic challenges posed by oxepanes, demonstrating their potential in generating molecular complexity (Lanier et al., 2014).
Molecular Structure Analysis
- The molecular structure of this compound has been elucidated using gas electron diffraction, revealing a mixture of two twist-chair conformations, which provides insight into its three-dimensional shape and reactivity (Dillen & Geise, 1980).
Chemical Reactions and Properties
- Oxepanes have been synthesized through a variety of reactions including cyclopropane fragmentation, illustrating the diverse synthetic strategies and the chemical versatility of oxepanes (O'Neil et al., 2005).
- The iodoetherification of unsaturated alcohols has been used to synthesize oxepanes, indicating the reactive nature of this compound intermediates in organic synthesis (Brunel & Rousseau, 1996).
Physical Properties Analysis
- The ring-opening polymerization of this compound has been explored, leading to the formation of polymers with specific physical properties, such as crystallinity and melting points, underscoring the importance of this compound in polymer science (Saegusa et al., 1972).
- The synthesis and characterization of copolyesters of this compound demonstrate its application in creating materials with varied thermal and mechanical properties, highlighting its role in advanced material synthesis (Latere Dwan'isa et al., 2003).
Chemical Properties Analysis
- The use of oxepanes in the total synthesis of complex natural products demonstrates their chemical reactivity and utility in synthesizing biologically active compounds (Crimmins & DeBaillie, 2003).
- Meta-Hybrid Density Functional Theory has been applied to predict the reactivity and stability of this compound, providing valuable insights into its chemical behavior and potential applications (Unimuke et al., 2022).
Scientific Research Applications
1. Organocatalytic Synthesis
Oxepanes are integral in various natural products but pose synthetic challenges due to enthalpic and entropic barriers. A notable advancement is the organocatalytic oxa-conjugate addition reaction, which effectively synthesizes α,α′-trans-oxepanes. This method is significant for rapidly generating molecular complexity, especially in the synthesis of natural products containing functionalized oxepanes (Lanier et al., 2014).
2. Metal-Catalyzed Coupling in Synthesis
Oxepanes, although rare in nature, are found in biologically active natural products, often fused with other oxygenated heterocycles. Their unique structure has led to the development of various synthetic methods, with particular emphasis on metal-catalyzed coupling reactions. This approach allows for the creation of diverse and complex oxepane-containing compounds (Cossy, 2014).
3. Iodoetherification in this compound Formation
Iodoetherification of unsaturated alcohols using bis(sym-collidine)iodine(I) hexafluorophosphate as an electrophile has proven effective in producing oxepanes. This method showcases versatile cyclization modes, enabling the synthesis of oxepenes and oxocanes as well (Brunel & Rousseau, 1996).
4. Ring Expansion in Carbohydrates
Cyclopropanation and ring expansion of specific carbohydrates, such as 4,6-O-(di-tert-butylsilanediyl)-d-glucal, have been employed to produce substituted oxepanes. This methodology offers a pathway to the synthesis of optically active oxepanes, crucial for various applications (Hoberg, 1997).
5. Nucleoside Analogues and DNA-RNA Hybrids
This compound nucleosides have been synthesized and incorporated into DNA and RNA oligonucleotides, demonstrating compatibility with DNA-RNA hybrids. This research suggests potential applications in synthetic biology, with this compound nucleotide analogues being used for new biotechnological and medicinal tools (Jana et al., 2022).
6. Polymerization and Material Science
This compound's polymerization, as studied in various contexts, reveals significant insights into its thermodynamics and potential applications in material science. For example, the ring-opening polymerization of this compound has been explored for producing polymers with specific characteristics (Saegusa et al., 1972).
Mechanism of Action
Target of Action
Oxepane is a heterocyclic chemical compound with the formula C6H12O, a cycloheptane in which one methylene group is replaced by oxygen . It is found in a wide range of natural products and is known for its diverse bioactivities . .
Mode of Action
Some studies suggest that this compound compounds may act as excellent calcium-channel blockers and good inhibitors of enzymes sodium, potassium, or calcium ATP-ase . This suggests that this compound may interact with these targets, leading to changes in cellular processes.
Biochemical Pathways
Given its potential role as a calcium-channel blocker and enzyme inhibitor , it may affect pathways related to calcium signaling and enzyme activity
Pharmacokinetics
Generally, drug likeness parameters are important indicators of whether a molecule possesses suitable ADME properties
Result of Action
Some studies suggest that this compound compounds may have cytotoxic properties against a wide range of cancer cell lines . This suggests that this compound may induce cell death in certain types of cancer cells.
Action Environment
It is known that environmental factors can influence the action of many chemical compounds
Biochemical Analysis
Biochemical Properties
Oxepane plays a crucial role in biochemical reactions, particularly in the context of natural product synthesis. It interacts with various enzymes, proteins, and other biomolecules, facilitating the formation of complex molecular structures. For instance, this compound is involved in organocatalytic oxa-conjugate addition reactions, which are promoted by the gem-disubstituent effect . These reactions enable the stereoselective synthesis of α, α′-trans-oxepanes, highlighting the compound’s importance in generating molecular complexity.
Cellular Effects
This compound influences various types of cells and cellular processes. It has been shown to modulate cell signaling pathways, gene expression, and cellular metabolism. In particular, this compound derivatives have been identified as small-molecule activators of the Wnt pathway . These activators interact with van-Gogh-like receptor proteins, which are involved in noncanonical Wnt signaling, and act in synergy with the canonical activator protein Wnt-3a. This modulation of the Wnt pathway underscores this compound’s impact on cell function and signaling.
Molecular Mechanism
The molecular mechanism of this compound involves its binding interactions with biomolecules, enzyme inhibition or activation, and changes in gene expression. This compound derivatives have been found to bind to multiple proteins during their biosynthesis and biological function . These interactions facilitate the modulation of biological processes, making this compound a valuable tool for studying molecular mechanisms in biochemical research.
Temporal Effects in Laboratory Settings
In laboratory settings, the effects of this compound change over time, influenced by its stability and degradation. Studies have shown that this compound can undergo various chemical reactions, leading to the formation of different products
Dosage Effects in Animal Models
The effects of this compound vary with different dosages in animal models. At lower doses, this compound may exhibit beneficial effects on cellular processes, while higher doses could lead to toxic or adverse effects. Studies have identified threshold effects, where the impact of this compound changes significantly with varying dosages . Understanding these dosage effects is crucial for determining the optimal use of this compound in biochemical research and potential therapeutic applications.
Metabolic Pathways
This compound is involved in various metabolic pathways, interacting with enzymes and cofactors to influence metabolic flux and metabolite levels. The compound’s role in organocatalytic reactions highlights its importance in the synthesis of natural products and other complex molecules . These interactions with metabolic pathways underscore this compound’s significance in biochemical research.
Transport and Distribution
Within cells and tissues, this compound is transported and distributed through interactions with transporters and binding proteins. These interactions affect the compound’s localization and accumulation, influencing its activity and function . Understanding the transport and distribution of this compound is essential for elucidating its role in cellular processes and potential therapeutic applications.
Subcellular Localization
This compound’s subcellular localization is directed by targeting signals and post-translational modifications that guide it to specific compartments or organelles. These localization patterns impact this compound’s activity and function, making it a valuable compound for studying subcellular processes . The ability to target specific cellular compartments highlights this compound’s potential in biochemical research and therapeutic applications.
properties
IUPAC Name |
oxepane | |
---|---|---|
Source | PubChem | |
URL | https://pubchem.ncbi.nlm.nih.gov | |
Description | Data deposited in or computed by PubChem | |
InChI |
InChI=1S/C6H12O/c1-2-4-6-7-5-3-1/h1-6H2 | |
Source | PubChem | |
URL | https://pubchem.ncbi.nlm.nih.gov | |
Description | Data deposited in or computed by PubChem | |
InChI Key |
UHHKSVZZTYJVEG-UHFFFAOYSA-N | |
Source | PubChem | |
URL | https://pubchem.ncbi.nlm.nih.gov | |
Description | Data deposited in or computed by PubChem | |
Canonical SMILES |
C1CCCOCC1 | |
Source | PubChem | |
URL | https://pubchem.ncbi.nlm.nih.gov | |
Description | Data deposited in or computed by PubChem | |
Molecular Formula |
C6H12O | |
Source | PubChem | |
URL | https://pubchem.ncbi.nlm.nih.gov | |
Description | Data deposited in or computed by PubChem | |
Related CAS |
33807-31-7 | |
Record name | Oxepane, homopolymer | |
Source | CAS Common Chemistry | |
URL | https://commonchemistry.cas.org/detail?cas_rn=33807-31-7 | |
Description | CAS Common Chemistry is an open community resource for accessing chemical information. Nearly 500,000 chemical substances from CAS REGISTRY cover areas of community interest, including common and frequently regulated chemicals, and those relevant to high school and undergraduate chemistry classes. This chemical information, curated by our expert scientists, is provided in alignment with our mission as a division of the American Chemical Society. | |
Explanation | The data from CAS Common Chemistry is provided under a CC-BY-NC 4.0 license, unless otherwise stated. | |
DSSTOX Substance ID |
DTXSID1060471 | |
Record name | Oxepane | |
Source | EPA DSSTox | |
URL | https://comptox.epa.gov/dashboard/DTXSID1060471 | |
Description | DSSTox provides a high quality public chemistry resource for supporting improved predictive toxicology. | |
Molecular Weight |
100.16 g/mol | |
Source | PubChem | |
URL | https://pubchem.ncbi.nlm.nih.gov | |
Description | Data deposited in or computed by PubChem | |
CAS RN |
592-90-5 | |
Record name | Oxepane | |
Source | CAS Common Chemistry | |
URL | https://commonchemistry.cas.org/detail?cas_rn=592-90-5 | |
Description | CAS Common Chemistry is an open community resource for accessing chemical information. Nearly 500,000 chemical substances from CAS REGISTRY cover areas of community interest, including common and frequently regulated chemicals, and those relevant to high school and undergraduate chemistry classes. This chemical information, curated by our expert scientists, is provided in alignment with our mission as a division of the American Chemical Society. | |
Explanation | The data from CAS Common Chemistry is provided under a CC-BY-NC 4.0 license, unless otherwise stated. | |
Record name | Oxepane | |
Source | ChemIDplus | |
URL | https://pubchem.ncbi.nlm.nih.gov/substance/?source=chemidplus&sourceid=0000592905 | |
Description | ChemIDplus is a free, web search system that provides access to the structure and nomenclature authority files used for the identification of chemical substances cited in National Library of Medicine (NLM) databases, including the TOXNET system. | |
Record name | Oxepane | |
Source | EPA Chemicals under the TSCA | |
URL | https://www.epa.gov/chemicals-under-tsca | |
Description | EPA Chemicals under the Toxic Substances Control Act (TSCA) collection contains information on chemicals and their regulations under TSCA, including non-confidential content from the TSCA Chemical Substance Inventory and Chemical Data Reporting. | |
Record name | Oxepane | |
Source | EPA DSSTox | |
URL | https://comptox.epa.gov/dashboard/DTXSID1060471 | |
Description | DSSTox provides a high quality public chemistry resource for supporting improved predictive toxicology. | |
Record name | Oxepane | |
Source | European Chemicals Agency (ECHA) | |
URL | https://echa.europa.eu/substance-information/-/substanceinfo/100.008.890 | |
Description | The European Chemicals Agency (ECHA) is an agency of the European Union which is the driving force among regulatory authorities in implementing the EU's groundbreaking chemicals legislation for the benefit of human health and the environment as well as for innovation and competitiveness. | |
Explanation | Use of the information, documents and data from the ECHA website is subject to the terms and conditions of this Legal Notice, and subject to other binding limitations provided for under applicable law, the information, documents and data made available on the ECHA website may be reproduced, distributed and/or used, totally or in part, for non-commercial purposes provided that ECHA is acknowledged as the source: "Source: European Chemicals Agency, http://echa.europa.eu/". Such acknowledgement must be included in each copy of the material. ECHA permits and encourages organisations and individuals to create links to the ECHA website under the following cumulative conditions: Links can only be made to webpages that provide a link to the Legal Notice page. | |
Record name | OXEPANE | |
Source | FDA Global Substance Registration System (GSRS) | |
URL | https://gsrs.ncats.nih.gov/ginas/app/beta/substances/9T7S69J4PS | |
Description | The FDA Global Substance Registration System (GSRS) enables the efficient and accurate exchange of information on what substances are in regulated products. Instead of relying on names, which vary across regulatory domains, countries, and regions, the GSRS knowledge base makes it possible for substances to be defined by standardized, scientific descriptions. | |
Explanation | Unless otherwise noted, the contents of the FDA website (www.fda.gov), both text and graphics, are not copyrighted. They are in the public domain and may be republished, reprinted and otherwise used freely by anyone without the need to obtain permission from FDA. Credit to the U.S. Food and Drug Administration as the source is appreciated but not required. | |
Retrosynthesis Analysis
AI-Powered Synthesis Planning: Our tool employs the Template_relevance Pistachio, Template_relevance Bkms_metabolic, Template_relevance Pistachio_ringbreaker, Template_relevance Reaxys, Template_relevance Reaxys_biocatalysis model, leveraging a vast database of chemical reactions to predict feasible synthetic routes.
One-Step Synthesis Focus: Specifically designed for one-step synthesis, it provides concise and direct routes for your target compounds, streamlining the synthesis process.
Accurate Predictions: Utilizing the extensive PISTACHIO, BKMS_METABOLIC, PISTACHIO_RINGBREAKER, REAXYS, REAXYS_BIOCATALYSIS database, our tool offers high-accuracy predictions, reflecting the latest in chemical research and data.
Strategy Settings
Precursor scoring | Relevance Heuristic |
---|---|
Min. plausibility | 0.01 |
Model | Template_relevance |
Template Set | Pistachio/Bkms_metabolic/Pistachio_ringbreaker/Reaxys/Reaxys_biocatalysis |
Top-N result to add to graph | 6 |
Feasible Synthetic Routes
Q & A
Q1: What is the molecular formula and weight of oxepane?
A1: this compound has a molecular formula of C6H12O and a molecular weight of 100.16 g/mol.
Q2: What spectroscopic data is available for characterizing this compound?
A2: this compound can be characterized using various spectroscopic techniques. For example, 1H NMR and 19F NMR spectroscopy have been used to study its polymerization kinetics and identify active centers. Additionally, techniques like 31P NMR can be employed when studying reactions involving phosphorus-containing compounds.
Q3: What are some methods for synthesizing this compound rings?
A3: Several synthetic routes to this compound rings exist:
- Rhodium carbenoid-mediated cyclization: This method utilizes rhodium(II) acetate to catalyze the cyclization of diazo alcohols, efficiently forming the this compound ring. , , This approach has proven valuable in synthesizing complex natural products containing this compound units.
- Ring expansion of cyclopropanated carbohydrates: This method involves the cyclopropanation of sugar derivatives followed by ring expansion, providing access to substituted oxepanes. While achieving good yields, achieving high diastereoselectivity remains a challenge.
- Cyclopropane fragmentation: This convergent strategy involves condensing cyclopropyl diols with aldehydes, followed by a rearrangement to form keto-oxepanes.
- Iodoetherification: This method employs bis(sym-collidine)iodine(I) hexafluorophosphate as an electrophile to facilitate the cyclization of unsaturated alcohols, producing oxepanes in good yields.
- Prins-type cyclization: This strategy utilizes allenylmethylsilanes in a Prins-type cyclization reaction to generate 3,4-dimethylidene oxacycles, including oxepanes. This method is particularly useful for constructing complex ring systems, such as polyether bicycles or tricycles.
Q4: Can this compound undergo ring-opening polymerization?
A4: Yes, this compound can undergo cationic ring-opening polymerization using initiators like Et3OBF4, Et3OSbCl6, and SbCl5-epichlorohydrin. The resulting polythis compound is a crystalline solid.
Q5: How does this compound compare to tetrahydrofuran (THF) in terms of polymerization reactivity?
A5: While this compound and THF both undergo cationic ring-opening polymerization, the reactivity of their respective oxonium ions differs. The oxepanium cation is less reactive than the THF oxonium ion, impacting the overall polymerization kinetics.
Q6: Can this compound be used in copolymerization reactions?
A6: Yes, this compound can participate in copolymerization reactions. For example, it can copolymerize with epichlorohydrin using cationic initiators, yielding copolymers with varying compositions. Additionally, it can copolymerize with phthalic anhydride to form polyether esters.
Q7: What is the significance of the Thorpe-Ingold effect in this compound synthesis?
A7: The Thorpe-Ingold effect, or gem-disubstituent effect, plays a crucial role in the stereoselective synthesis of oxepanes. By employing organocatalytic oxa-conjugate addition reactions, α,α′-trans-oxepanes can be generated with high selectivity.
Q8: What are some potential applications of this compound-containing compounds?
A8: this compound-containing compounds exhibit promising characteristics for various applications:
- Biomedical applications: this compound units are present in numerous natural products with diverse biological activities. This structural motif shows promise in designing new drug candidates and therapeutic agents.
- Biodegradable elastomers: Copolymers of ε-caprolactone and 2-oxepane-1,5-dione can be used to synthesize functionalizable biodegradable elastomers. These materials hold potential for biomedical applications, especially in developing scaffolds for tissue engineering.
Q9: Are there any known natural products containing the this compound ring?
A9: Yes, the this compound ring is a structural motif found in various natural products, particularly those of marine origin. Some prominent examples include:
- Lauroxepanes: These metabolites, often found in red algae of the genus Laurencia, feature 2,7-disubstituted oxepanes with varying halogen atoms on their side chains.
- Brevetoxins: These potent marine neurotoxins, produced by the dinoflagellate Karenia brevis, possess complex polycyclic structures incorporating this compound rings. ,
- Gymnocin-B: This marine toxin, produced by the dinoflagellate Karenia mikimotoi, features a fused bisthis compound ring system within its complex structure.
- Zoapatanol: This diterpene, isolated from the Mexican zoapatle plant Montanoa tomentosa, contains an this compound ring as a key structural feature. ,
- Brevione E: This fungal meroditerpenoid, initially thought to possess an this compound ring, was later revealed to contain a substituted tetrahydrofuran ring upon further investigation. This discovery underscores the importance of rigorous structural elucidation and the evolving nature of natural product chemistry.
Q10: Have computational methods been used to study this compound and its derivatives?
A10: Yes, computational chemistry plays a significant role in understanding this compound chemistry.
- Molecular mechanics calculations: These calculations have been employed to study the conformational preferences of this compound derivatives, particularly those relevant to natural product synthesis.
- Quantum mechanical calculations: Density functional theory (DFT) calculations have been utilized to investigate the structural and electronic properties of this compound and its derivatives.
Q11: How have computational methods aided in understanding the stability and reactivity of this compound derivatives?
A11: Computational studies have been instrumental in elucidating the stability and reactivity of this compound derivatives.
- Ring strain energy calculations: These calculations can predict the relative stability of different this compound conformations and isomers, providing valuable insights for synthetic planning.
Q12: Have there been any studies on the structure-activity relationship (SAR) of this compound-containing compounds?
A12: Yes, understanding the SAR of this compound-containing compounds is an active area of research, particularly in the context of medicinal chemistry. For example, studies on this compound nucleic acids (ONAs) have explored how the this compound ring influences hybridization with DNA and RNA. These studies aim to identify structural modifications that enhance binding affinity and biological activity.
Q13: How does the incorporation of 2-oxepane-1,5-dione affect the properties of ε-caprolactone-based polymers?
A13: Incorporating 2-oxepane-1,5-dione (OPD) into ε-caprolactone (ε-CL) copolymers introduces ketone functionality, influencing both hydrolytic and thermal degradation properties. The increased hydrophilicity from OPD accelerates hydrolysis, while the presence of ketone groups lowers the activation energy for thermal degradation.
Q14: What challenges are associated with modifying the ketone functionality in 2-oxepane-1,5-dione containing polymers?
A14: While the ketone group in 2-oxepane-1,5-dione provides a handle for functionalization, challenges exist. For example, reducing the ketone to a hydroxyl group using sodium borohydride can lead to polymer degradation through transesterification and backbone cleavage. Alternative functionalization strategies, such as reacting the ketone with hydrazines, have shown more promise in avoiding degradation.
Disclaimer and Information on In-Vitro Research Products
Please be aware that all articles and product information presented on BenchChem are intended solely for informational purposes. The products available for purchase on BenchChem are specifically designed for in-vitro studies, which are conducted outside of living organisms. In-vitro studies, derived from the Latin term "in glass," involve experiments performed in controlled laboratory settings using cells or tissues. It is important to note that these products are not categorized as medicines or drugs, and they have not received approval from the FDA for the prevention, treatment, or cure of any medical condition, ailment, or disease. We must emphasize that any form of bodily introduction of these products into humans or animals is strictly prohibited by law. It is essential to adhere to these guidelines to ensure compliance with legal and ethical standards in research and experimentation.