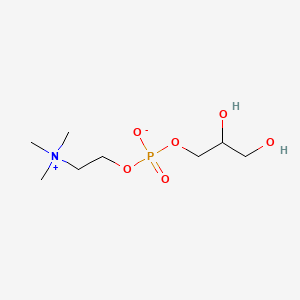
Glycerylphosphorylcholine
Overview
Description
Glycerylphosphorylcholine is a naturally occurring choline compound found in the brain and other tissues. It is a subclass of glycerophospholipids containing choline as the headgroup. The quarternary nitrogen of the choline group carries a permanent positive charge, while the phosphate group is deprotonated at physiological pH values, giving a net charge of zero . This compound is a major constituent of cell membranes and plays a crucial role in maintaining cell-membrane integrity .
Mechanism of Action
Target of Action
Glycerophosphocholine (GPC) is a bioactive, diffusible phosphoinositide metabolite that acts both intracellularly and in a paracrine fashion . It interacts with various targets, including immune cells , and plays a role in modulating T-cell signaling . GPC can also interact with intracellular targets and/or be released into the extracellular medium through specific membrane transporters .
Mode of Action
GPC’s mode of action involves its interaction with its targets and the resulting changes. For instance, it has been shown that exogenous GPC enhances CXCL12-induced T-cell chemotaxis through activation of the kinase Lck in a cAMP/PKA-dependent manner . This highlights the potential of GPC as an immunomodulator .
Biochemical Pathways
GPC is involved in several biochemical pathways. It is a metabolite of phospholipase A2 and is generated from membrane phosphoinositides through two sequential deacylation reactions . GPC can activate the IIS pathway by regulating the expression of daf-2, ins-18, and daf-16 genes, sek-1 and skn-1 genes of the MAPK pathway, and sod-3, ctl-1, gst-4, and other antioxidant genes .
Pharmacokinetics
GPC is a major glycerophospholipid in eukaryotic cellular membranes . It is synthesized through two different pathways: the CDP-choline pathway (also known as the Kennedy pathway) and the PEMT pathway . The CDP-choline pathway involves the phosphorylation of choline by choline kinase, while the PEMT pathway involves the sequential methylation of glycerophosphoethanolamine . The PEMT pathway contributes to about 30% of hepatic GPC .
Result of Action
The molecular and cellular effects of GPC’s action are diverse. It participates in central processes, including cell proliferation and survival . GPC can also control actin dynamics in several cell systems by regulating Rho GTPases . Moreover, it has been shown to have an anti-aging effect in certain organisms .
Action Environment
Environmental factors can influence GPC’s action, efficacy, and stability. For instance, GPC can relieve symptoms related to aging in organisms . .
Biochemical Analysis
Biochemical Properties
Glycerophosphocholine plays a crucial role in various biochemical reactions. It is involved in the synthesis of acetylcholine, which is essential for neurotransmission. The compound interacts with enzymes such as choline acetyltransferase, which catalyzes the formation of acetylcholine from choline and acetyl-CoA. Additionally, glycerophosphocholine is metabolized by glycerophosphocholine phosphodiesterase, which hydrolyzes it into choline and glycerol-3-phosphate .
Cellular Effects
Glycerophosphocholine has significant effects on various cell types and cellular processes. It supports cellular membranes and enhances cell signaling pathways. In neurons, glycerophosphocholine increases the synthesis and release of acetylcholine, which is involved in memory, motivation, and attention. It also influences gene expression and cellular metabolism by providing choline for the synthesis of phosphatidylcholine, a major component of cell membranes .
Molecular Mechanism
At the molecular level, glycerophosphocholine exerts its effects by increasing the availability of choline, which is a precursor for acetylcholine synthesis. The compound crosses the blood-brain barrier and is rapidly absorbed by neuronal cells. It binds to choline transporters and is taken up into the cells, where it is converted into acetylcholine. This process involves the enzyme choline acetyltransferase, which transfers an acetyl group from acetyl-CoA to choline, forming acetylcholine .
Temporal Effects in Laboratory Settings
In laboratory settings, the effects of glycerophosphocholine have been observed to change over time. The compound is stable under physiological conditions and does not degrade rapidly. Long-term studies have shown that glycerophosphocholine intake can suppress microglial activation and blood-brain barrier disruption, promoting neurogenesis and sustaining cellular function in the brain .
Dosage Effects in Animal Models
The effects of glycerophosphocholine vary with different dosages in animal models. Studies have shown that higher doses of glycerophosphocholine can enhance cognitive function and neuroprotection. Extremely high doses may lead to adverse effects such as increased risk of stroke. In animal models, a dosage of 300-600 mg/kg has been found to be effective without causing significant toxicity .
Metabolic Pathways
Glycerophosphocholine is involved in several metabolic pathways It is metabolized into choline and glycerol-3-phosphate by glycerophosphocholine phosphodiesteraseThis pathway involves the enzymes choline kinase, CTP:phosphocholine cytidylyltransferase, and CDP-choline:1,2-diacylglycerol cholinephosphotransferase .
Transport and Distribution
Glycerophosphocholine is transported and distributed within cells and tissues through specific transporters and binding proteins. It crosses the blood-brain barrier and is taken up by neuronal cells via choline transporters. Once inside the cells, glycerophosphocholine is distributed to various cellular compartments, including the cytosol and mitochondria, where it participates in metabolic processes .
Subcellular Localization
The subcellular localization of glycerophosphocholine is primarily in the cytosol and mitochondria. It is involved in the synthesis of phosphatidylcholine, which is a major component of cellular membranes. Glycerophosphocholine is also found in the perinuclear region, where it interacts with enzymes involved in phospholipid metabolism. The compound’s localization is regulated by specific targeting signals and post-translational modifications .
Preparation Methods
Glycerylphosphorylcholine can be prepared through various methods. One common method involves the transesterification of phosphatidylcholine catalyzed by low boiling point amines under mild conditions . This process uses catalysts such as isopropylamine, which shows high activity and yields . Another method involves the hydrolysis of natural soybean or egg yolk lecithin, which is more suitable for producing food-grade glycerophosphocholine . Industrially, it can also be produced by the chemical or enzymatic deacylation of phosphatidylcholine enriched soya phospholipids followed by chromatographic purification .
Chemical Reactions Analysis
Glycerylphosphorylcholine undergoes various chemical reactions, including oxidation, reduction, and substitution. It can be hydrolyzed by phospholipases to produce monoacylglycerophosphocholine . In the gastrointestinal tract, it metabolizes to trimethylamine n-oxide, which has implications for cardiovascular health . Common reagents used in these reactions include phospholipases and low boiling point amines . Major products formed from these reactions include monoacylglycerophosphocholine and trimethylamine n-oxide .
Scientific Research Applications
Glycerylphosphorylcholine has numerous scientific research applications. In medicine, it is investigated for its potential in treating Alzheimer’s disease and other dementias due to its role as a precursor of acetylcholine . It is also used as a dietary supplement to enhance cognitive function and motivation . In biology, it plays a role in maintaining cell-membrane integrity and fluidity . Additionally, it is used in the pharmaceutical field for its capability of incorporating other active ingredients and intrinsic therapeutic activities .
Comparison with Similar Compounds
Glycerylphosphorylcholine is often compared with other choline compounds such as citicoline (CDP Choline) and phosphatidylcholine. Citicoline is a naturally occurring compound found in every cell of the body and is an essential intermediary in the synthesis of phosphatidylcholine . Phosphatidylcholine is a major constituent of cell membranes and plays a role in maintaining cell-membrane integrity . While both citicoline and glycerophosphocholine are significant sources of choline, they differ in their mechanisms of action, metabolic pathways, and bioavailability . This compound is unique in its ability to rapidly deliver choline to the brain and enhance cholinergic transmission .
Properties
IUPAC Name |
2-[2,3-dihydroxypropoxy(hydroxy)phosphoryl]oxyethyl-trimethylazanium | |
---|---|---|
Details | Computed by Lexichem TK 2.7.0 (PubChem release 2021.10.14) | |
Source | PubChem | |
URL | https://pubchem.ncbi.nlm.nih.gov | |
Description | Data deposited in or computed by PubChem | |
InChI |
InChI=1S/C8H20NO6P/c1-9(2,3)4-5-14-16(12,13)15-7-8(11)6-10/h8,10-11H,4-7H2,1-3H3/p+1 | |
Details | Computed by InChI 1.0.6 (PubChem release 2021.10.14) | |
Source | PubChem | |
URL | https://pubchem.ncbi.nlm.nih.gov | |
Description | Data deposited in or computed by PubChem | |
InChI Key |
SUHOQUVVVLNYQR-UHFFFAOYSA-O | |
Details | Computed by InChI 1.0.6 (PubChem release 2021.10.14) | |
Source | PubChem | |
URL | https://pubchem.ncbi.nlm.nih.gov | |
Description | Data deposited in or computed by PubChem | |
Canonical SMILES |
C[N+](C)(C)CCOP(=O)(O)OCC(CO)O | |
Details | Computed by OEChem 2.3.0 (PubChem release 2021.10.14) | |
Source | PubChem | |
URL | https://pubchem.ncbi.nlm.nih.gov | |
Description | Data deposited in or computed by PubChem | |
Molecular Formula |
C8H21NO6P+ | |
Details | Computed by PubChem 2.2 (PubChem release 2021.10.14) | |
Source | PubChem | |
URL | https://pubchem.ncbi.nlm.nih.gov | |
Description | Data deposited in or computed by PubChem | |
DSSTOX Substance ID |
DTXSID40862200 | |
Record name | 2-{[(2,3-Dihydroxypropoxy)(hydroxy)phosphoryl]oxy}-N,N,N-trimethylethan-1-aminium | |
Source | EPA DSSTox | |
URL | https://comptox.epa.gov/dashboard/DTXSID40862200 | |
Description | DSSTox provides a high quality public chemistry resource for supporting improved predictive toxicology. | |
Molecular Weight |
258.23 g/mol | |
Details | Computed by PubChem 2.2 (PubChem release 2021.10.14) | |
Source | PubChem | |
URL | https://pubchem.ncbi.nlm.nih.gov | |
Description | Data deposited in or computed by PubChem | |
CAS No. |
563-24-6 | |
Record name | 2-{[(2,3-Dihydroxypropoxy)(hydroxy)phosphoryl]oxy}-N,N,N-trimethylethan-1-aminium | |
Source | EPA DSSTox | |
URL | https://comptox.epa.gov/dashboard/DTXSID40862200 | |
Description | DSSTox provides a high quality public chemistry resource for supporting improved predictive toxicology. | |
Retrosynthesis Analysis
AI-Powered Synthesis Planning: Our tool employs the Template_relevance Pistachio, Template_relevance Bkms_metabolic, Template_relevance Pistachio_ringbreaker, Template_relevance Reaxys, Template_relevance Reaxys_biocatalysis model, leveraging a vast database of chemical reactions to predict feasible synthetic routes.
One-Step Synthesis Focus: Specifically designed for one-step synthesis, it provides concise and direct routes for your target compounds, streamlining the synthesis process.
Accurate Predictions: Utilizing the extensive PISTACHIO, BKMS_METABOLIC, PISTACHIO_RINGBREAKER, REAXYS, REAXYS_BIOCATALYSIS database, our tool offers high-accuracy predictions, reflecting the latest in chemical research and data.
Strategy Settings
Precursor scoring | Relevance Heuristic |
---|---|
Min. plausibility | 0.01 |
Model | Template_relevance |
Template Set | Pistachio/Bkms_metabolic/Pistachio_ringbreaker/Reaxys/Reaxys_biocatalysis |
Top-N result to add to graph | 6 |
Feasible Synthetic Routes
Disclaimer and Information on In-Vitro Research Products
Please be aware that all articles and product information presented on BenchChem are intended solely for informational purposes. The products available for purchase on BenchChem are specifically designed for in-vitro studies, which are conducted outside of living organisms. In-vitro studies, derived from the Latin term "in glass," involve experiments performed in controlled laboratory settings using cells or tissues. It is important to note that these products are not categorized as medicines or drugs, and they have not received approval from the FDA for the prevention, treatment, or cure of any medical condition, ailment, or disease. We must emphasize that any form of bodily introduction of these products into humans or animals is strictly prohibited by law. It is essential to adhere to these guidelines to ensure compliance with legal and ethical standards in research and experimentation.