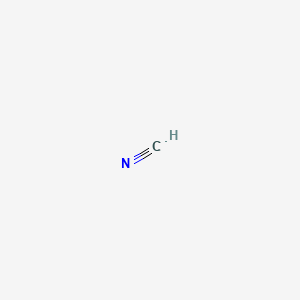
Hydrogen cyanide
Overview
Description
Hydrogen Cyanide is a highly toxic conjugate acid of a cyanide that is used as a chemical weapon agent. It is characterized as a colorless gas or liquid with a strong pungent odor that causes irritation of the eyes and respiratory tract, as well as toxic systemic effects.
This compound is a colorless or pale-blue liquid (hydrocyanic acid); at higher temperatures, it is a colorless gas. This compound is very volatile, producing potentially lethal concentrations at room temperature. The vapor is flammable and potentially explosive. This compound has a faint, bitter almond odor and a bitter, burning taste. It is soluble in water and is often used as a 96% aqueous solution.
This compound, anhydrous, stabilized (absorbed) appears as a clear colorless liquid with a faint odor of bitter almonds that is absorbed in a porous inert material. Absorption slows evolution of vapors. Vapors slightly lighter than air. Deadly poison by all routes (absorption through skin of liquid, inhalation of vapors, etc). Prolonged exposure of closed containers to heat may cause violent rupture and rocketing. Rate of onset: Immediate Persistence: Minutes Odor threshold: 1-5 ppm Source/use/other hazard: War gas, pesticide, Herbicide; other industries; Weak acid except in water or mucous membranes - then corrosive/training.
Properties
CAS No. |
74-90-8 |
---|---|
Molecular Formula |
HCN CHN |
Molecular Weight |
27.025 g/mol |
IUPAC Name |
formonitrile |
InChI |
InChI=1S/CHN/c1-2/h1H |
InChI Key |
LELOWRISYMNNSU-UHFFFAOYSA-N |
SMILES |
C#N |
Canonical SMILES |
C#N |
boiling_point |
78.1 °F at 760 mm Hg (EPA, 1998) 78 °F at 760 mm Hg (96%) (NIOSH, 2016) 25.6 °C 25.63 °C 26 °C 78°F (96%) |
Color/Form |
Colorless gas or liquid Water-white liquid below 26.5 °C Colorless or pale-blue liquid or gas (above 78 °F) ... [Note: Often used as a 96% solution in water] |
density |
0.699 (EPA, 1998) 0.69 (NIOSH, 2016) 0.6875 g/cu cm at 20 °C Relative density (water = 1): 0.69 (liquid) 0.69 |
flash_point |
0 °F (EPA, 1998) 0 °F (96%) (NIOSH, 2016) 0 °F (-18 °C) (Closed cup) -18 °C c.c. 0°F (96%) |
melting_point |
7.9 °F (EPA, 1998) 7 °F (96%) (NIOSH, 2016) -13.4 °C -13.28 °C -13 °C 7°F (96%) |
74-90-8 | |
physical_description |
Hydrocyanic acid, aqueous solution, with not more than 20% hydrogen cyanide is a clear colorless aqueous solution of a gas. Has a faint odor of almonds. Can evolve hydrogen cyanide gas, which is (barely) lighter than air. Flame can flash back to the source of a gas leak very easily. Lethal doses of gas may be inhaled. Lethal doses of cyanide can be absorbed from the solution through the skin. Hydrocyanic acid, aqueous solutions, with more than 20% hydrogen cyanide is a clear colorless aqueous solution of a gas. Has an odor of almonds. Can evolve hydrogen cyanide gas, which is (barely) lighter than air. Flame can flash back to the source of a gas leak very easily. Lethal doses of gas may be inhaled. Lethal doses of cyanide can be absorbed from the solution through the skin. Hydrocyanic acid, liquefied appears as a clear colorless liquid with a faint odor of bitter almonds. Boiling point 78°F. Density 5.7 lb / gal. Flash point 0°F. Evaporates easily (or boils) at room temperature. Vapors slightly lighter than air. Deadly poison by all routes (absorption through skin of liquid, inhalation of vapors, etc). Prolonged exposure of closed containers to heat may cause violent rupture and rocketing. Hydrogen cyanide, anhydrous, stabilized appears as very volatile colorless or pale-blue liquid or gas (above 78°F) with a bitter, almond-like odor. Often used as a 96% solution in water. (NIOSH, 2016) A deadly human poison by all routes. Hydrogen cyanide, anhydrous, stabilized (absorbed) appears as a clear colorless liquid with a faint odor of bitter almonds that is absorbed in a porous inert material. Absorption slows evolution of vapors. Vapors slightly lighter than air. Deadly poison by all routes (absorption through skin of liquid, inhalation of vapors, etc). Prolonged exposure of closed containers to heat may cause violent rupture and rocketing. Rate of onset: Immediate Persistence: Minutes Odor threshold: 1-5 ppm Source/use/other hazard: War gas, pesticide, Herbicide; other industries; Weak acid except in water or mucous membranes - then corrosive/training. GasVapor; GasVapor, Liquid; Liquid COLOURLESS GAS OR LIQUID WITH CHARACTERISTIC ODOUR. Colorless or pale-blue liquid or gas (above 78°F) with a bitter, almond-like odor. Colorless or pale-blue liquid or gas (above 78°F) with a bitter, almond-like odor. [Note: Often used as a 96% solution in water.] Colorless or pale blue liquid below 78°F (25.6°C), colorless gas above 78°F (25.6°C). |
Pictograms |
Flammable; Acute Toxic; Environmental Hazard |
shelf_life |
Soln sensitive to light. Anhydrous hydrogen cyanide is stable at or below room temperature if inhibited with acid (eg., 0.1% sulfuric acid). |
solubility |
Miscible (NIOSH, 2016) 37.00 M Miscible with water Miscible with ethanol, ethyl ether Miscible with alcohol; slightly soluble in ether Solubility in water: miscible Miscible |
Synonyms |
Acid, Hydrocyanic Cyanide, Hydrogen Hydrocyanic Acid Hydrogen Cyanide Zyklon B |
vapor_density |
0.901 (EPA, 1998) (Relative to Air) 0.932 (Air = 1) Relative vapor density (air = 1): 0.94 |
vapor_pressure |
630 mm Hg (EPA, 1998) 630 mm Hg (NIOSH, 2016) 741.82 mmHg 742 mm Hg at 25 °C Vapor pressure, kPa at 20 °C: 82.6 630 mmHg |
Origin of Product |
United States |
Synthesis routes and methods I
Procedure details
Synthesis routes and methods II
Procedure details
Synthesis routes and methods III
Procedure details
Retrosynthesis Analysis
AI-Powered Synthesis Planning: Our tool employs the Template_relevance Pistachio, Template_relevance Bkms_metabolic, Template_relevance Pistachio_ringbreaker, Template_relevance Reaxys, Template_relevance Reaxys_biocatalysis model, leveraging a vast database of chemical reactions to predict feasible synthetic routes.
One-Step Synthesis Focus: Specifically designed for one-step synthesis, it provides concise and direct routes for your target compounds, streamlining the synthesis process.
Accurate Predictions: Utilizing the extensive PISTACHIO, BKMS_METABOLIC, PISTACHIO_RINGBREAKER, REAXYS, REAXYS_BIOCATALYSIS database, our tool offers high-accuracy predictions, reflecting the latest in chemical research and data.
Strategy Settings
Precursor scoring | Relevance Heuristic |
---|---|
Min. plausibility | 0.01 |
Model | Template_relevance |
Template Set | Pistachio/Bkms_metabolic/Pistachio_ringbreaker/Reaxys/Reaxys_biocatalysis |
Top-N result to add to graph | 6 |
Feasible Synthetic Routes
Disclaimer and Information on In-Vitro Research Products
Please be aware that all articles and product information presented on BenchChem are intended solely for informational purposes. The products available for purchase on BenchChem are specifically designed for in-vitro studies, which are conducted outside of living organisms. In-vitro studies, derived from the Latin term "in glass," involve experiments performed in controlled laboratory settings using cells or tissues. It is important to note that these products are not categorized as medicines or drugs, and they have not received approval from the FDA for the prevention, treatment, or cure of any medical condition, ailment, or disease. We must emphasize that any form of bodily introduction of these products into humans or animals is strictly prohibited by law. It is essential to adhere to these guidelines to ensure compliance with legal and ethical standards in research and experimentation.