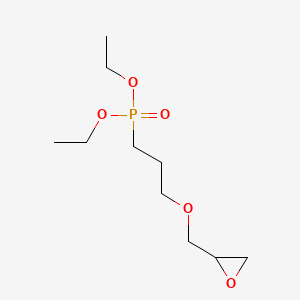
Diethyl (3-(oxiranylmethoxy)propyl)phosphonate
- Click on QUICK INQUIRY to receive a quote from our team of experts.
- With the quality product at a COMPETITIVE price, you can focus more on your research.
Overview
Description
Diethyl (3-(oxiranylmethoxy)propyl)phosphonate is an organic compound with the molecular formula C10H21O5P It contains a phosphonate group, which is known for its stability and resistance to hydrolysis
Preparation Methods
The synthesis of diethyl (3-(oxiranylmethoxy)propyl)phosphonate can be achieved through several methods. One common approach involves the Michaelis–Arbuzov reaction, where trialkyl phosphites react with alkyl halides . Another method includes the acylation of methylphosphonates, which involves metalation followed by reaction with acetyl chloride . Industrial production methods often rely on these reactions due to their efficiency and scalability.
Chemical Reactions Analysis
Diethyl (3-(oxiranylmethoxy)propyl)phosphonate undergoes various chemical reactions, including:
Substitution Reactions: It can participate in nucleophilic substitution reactions, where the phosphonate group is replaced by other nucleophiles.
Oxidation and Reduction: The compound can be oxidized or reduced under specific conditions, leading to different products.
Cross-Coupling Reactions: Using catalysts like palladium, it can undergo cross-coupling reactions with aryl and vinyl halides.
Common reagents used in these reactions include triflic anhydride for activation and various nucleophiles for substitution . Major products formed depend on the specific reaction conditions and reagents used.
Scientific Research Applications
Diethyl (3-(oxiranylmethoxy)propyl)phosphonate has several scientific research applications:
Organic Synthesis: It is used as a building block in the synthesis of complex organic molecules.
Medicinal Chemistry: The compound’s stability and resistance to hydrolysis make it a valuable component in drug development.
Industrial Applications: It is used in the production of agrochemicals and other industrial chemicals.
Mechanism of Action
The mechanism of action of diethyl (3-(oxiranylmethoxy)propyl)phosphonate involves its interaction with various molecular targets. The phosphonate group can form stable complexes with metal ions, influencing biochemical pathways . Additionally, its resistance to hydrolysis allows it to remain active in biological systems for extended periods .
Comparison with Similar Compounds
Properties
CAS No. |
55884-03-2 |
---|---|
Molecular Formula |
C10H21O5P |
Molecular Weight |
252.24 g/mol |
IUPAC Name |
2-(3-diethoxyphosphorylpropoxymethyl)oxirane |
InChI |
InChI=1S/C10H21O5P/c1-3-14-16(11,15-4-2)7-5-6-12-8-10-9-13-10/h10H,3-9H2,1-2H3 |
InChI Key |
PCAJGNDUGRGSNK-UHFFFAOYSA-N |
Canonical SMILES |
CCOP(=O)(CCCOCC1CO1)OCC |
Origin of Product |
United States |
Disclaimer and Information on In-Vitro Research Products
Please be aware that all articles and product information presented on BenchChem are intended solely for informational purposes. The products available for purchase on BenchChem are specifically designed for in-vitro studies, which are conducted outside of living organisms. In-vitro studies, derived from the Latin term "in glass," involve experiments performed in controlled laboratory settings using cells or tissues. It is important to note that these products are not categorized as medicines or drugs, and they have not received approval from the FDA for the prevention, treatment, or cure of any medical condition, ailment, or disease. We must emphasize that any form of bodily introduction of these products into humans or animals is strictly prohibited by law. It is essential to adhere to these guidelines to ensure compliance with legal and ethical standards in research and experimentation.