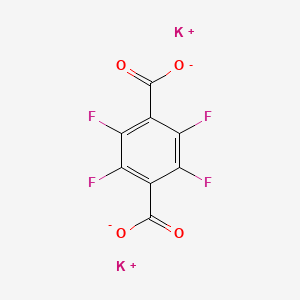
Potassium tetrafluoroterephthalate
- Click on QUICK INQUIRY to receive a quote from our team of experts.
- With the quality product at a COMPETITIVE price, you can focus more on your research.
Overview
Description
Potassium tetrafluoroterephthalate (K₂C₈F₄O₄) is a fluorinated carboxylate salt derived from tetrafluoroterephthalic acid. It serves as a critical ligand in coordination chemistry and materials science, particularly in constructing metal-organic frameworks (MOFs) and luminescent lanthanide complexes. The compound features two carboxylate groups and four fluorine substituents symmetrically positioned on the benzene ring, enhancing its electron-withdrawing properties and steric rigidity .
Synthesis and Structural Features:
this compound is typically synthesized via deprotonation of tetrafluoroterephthalic acid using potassium hydroxide. Its crystal structure often involves coordination to metal ions (e.g., Zn²⁺, Cd²⁺, Er³⁺) through carboxylate oxygen atoms, forming extended networks or discrete complexes. For example, in erbium-based MOFs, the ligand facilitates strong near-infrared (NIR) luminescence due to reduced vibrational quenching from C–H bonds .
Preparation Methods
Synthetic Routes and Reaction Conditions: Potassium tetrafluoroterephthalate can be synthesized by reacting tetrafluoroterephthalic acid with potassium hydroxide. The reaction typically occurs in an aqueous medium, and the product is isolated by crystallization.
Industrial Production Methods: In industrial settings, the production of this compound involves the use of high-purity reagents and controlled reaction conditions to ensure the consistency and quality of the final product. The process may include steps such as purification, drying, and packaging to meet industrial standards.
Chemical Reactions Analysis
Types of Reactions: Potassium tetrafluoroterephthalate undergoes various chemical reactions, including:
Substitution Reactions: It can participate in nucleophilic substitution reactions where the fluorine atoms are replaced by other nucleophiles.
Coordination Reactions: It forms coordination complexes with metal ions, which can lead to the formation of supramolecular structures.
Common Reagents and Conditions:
Nucleophiles: Common nucleophiles used in substitution reactions include amines and thiols.
Metal Ions: Metal ions such as scandium, zinc, and lanthanides are used in coordination reactions.
Major Products Formed:
Substitution Products: Depending on the nucleophile, various substituted terephthalates can be formed.
Coordination Complexes: These complexes often exhibit unique photoluminescent properties.
Scientific Research Applications
Chemistry
Ligand in Coordination Chemistry
Potassium tetrafluoroterephthalate serves as a ligand in the synthesis of coordination polymers and metal-organic frameworks (MOFs). The presence of fluorine atoms enhances the stability and reactivity of the resulting complexes. For instance, research has shown that fluorinated MOFs exhibit improved gas adsorption properties, particularly for hydrogen and carbon dioxide. The enthalpy of hydrogen adsorption in certain fluorinated MOFs was reported to be significantly higher than in non-fluorinated counterparts, indicating enhanced performance for applications in gas storage and separation technologies .
Synthesis of Advanced Materials
The compound is also utilized in the development of advanced materials with specific properties such as photoluminescence and thermal stability. Its ability to form stable coordination complexes makes it suitable for creating materials that can withstand extreme conditions while maintaining their structural integrity.
Biology
Biological Activity Studies
Derivatives of this compound are being investigated for potential biological activities. Research has indicated that these derivatives may exhibit antimicrobial properties, making them candidates for further exploration in pharmaceutical applications. The unique chemical structure allows for interactions with biological targets, which could lead to the development of new therapeutic agents.
Medicine
Drug Delivery Systems
Ongoing research is exploring the use of this compound in drug delivery systems. Its chemical properties may facilitate the transport of therapeutic compounds within biological systems, enhancing their efficacy and bioavailability. This application is particularly relevant in targeted therapies where precise delivery is crucial.
Industrial Applications
Production of Specialty Chemicals
This compound is involved in the production of specialty chemicals used in various industrial processes. For example, it has been identified as a synthetic intermediate for agrichemicals, highlighting its versatility in chemical manufacturing . The compound's stability under different conditions makes it an attractive option for industries seeking reliable raw materials.
Data Tables
Application | Description | Key Benefits |
---|---|---|
Coordination Chemistry | Used as a ligand in coordination polymers and MOFs | Enhanced stability and gas adsorption |
Advanced Materials | Development of materials with photoluminescence and thermal stability | High performance under extreme conditions |
Biological Activity | Potential antimicrobial properties of derivatives | Novel therapeutic agents |
Drug Delivery Systems | Facilitates transport of therapeutic compounds | Improved efficacy and bioavailability |
Specialty Chemicals | Synthetic intermediate for agrichemicals | Versatile application in chemical manufacturing |
Case Studies
Case Study 1: Coordination Polymers
A study published in Transition Metal Chemistry detailed the synthesis of cobalt(II) coordination polymers using this compound as a ligand. The resulting polymers exhibited unique structural properties and enhanced gas adsorption capabilities, demonstrating the compound's effectiveness in creating functional materials .
Case Study 2: Drug Delivery Research
Research conducted at a leading pharmaceutical university investigated the use of this compound derivatives in drug delivery systems. The study found that these derivatives could significantly improve the solubility and stability of poorly soluble drugs, leading to better therapeutic outcomes.
Mechanism of Action
The mechanism by which potassium tetrafluoroterephthalate exerts its effects is primarily through its ability to form stable coordination complexes with metal ions. These complexes can interact with various molecular targets and pathways, leading to unique chemical and physical properties. The presence of fluorine atoms enhances the compound’s reactivity and stability.
Comparison with Similar Compounds
Structural and Electronic Effects
Tetrafluoroterephthalate vs. Terephthalate (BDC)
Key Findings :
- Fluorination increases the ligand’s triplet state energy, enabling better energy transfer to lanthanide ions like Er³⁺ .
- In MOFs, fluorine substituents enhance Lewis acidity of metal nodes (e.g., Zr⁴⁺), improving catalytic activity for reactions like CO₂ adsorption .
Tetrafluoroterephthalate vs. Nitroterephthalate (NO₂-BDC)
Key Findings :
- Fluorine’s stronger electron-withdrawing effect compared to –NO₂ increases MOF node acidity, favoring catalytic applications .
- Fluorinated MOFs exhibit superior moisture stability in adsorption processes .
Luminescence Performance
Tetrafluoroterephthalate vs. Camphorate
Property | Tetrafluoroterephthalate | Camphorate | Reference |
---|---|---|---|
Triplet State Energy | 24,700 cm⁻¹ | 23,700 cm⁻¹ | |
Energy Transfer | Low efficiency for Pr³⁺/Nd³⁺ | Similar inefficiency | |
Ligand Type | Aromatic | Aliphatic |
Key Findings :
- Both ligands show poor energy transfer to Pr³⁺ and Nd³⁺ due to mismatched energy levels, limiting their use as "antenna" ligands .
- Tetrafluoroterephthalate’s rigid aromatic structure provides better framework stability in lanthanide MOFs compared to flexible camphorate .
Adsorption and Catalytic Properties
Fluorinated vs. Non-Fluorinated MOFs
Key Findings :
- Fluorination improves CO₂ uptake in humid conditions by reducing water competition in pores .
- Ce-based F4_MIL-140A exhibits unique ultramicroporous channels lined with fluorine atoms, enhancing selectivity for small gas molecules .
Metal Coordination Diversity
Zinc and Cobalt Complexes
Complex | Ligand | Luminescence | Reference |
---|---|---|---|
[Zn(H₂O)₆][Zn(H₂O)₄(L2)₂]·H₂O | Octafluorobiphenyl-4,4′-dicarboxylate | Visible emission | |
[Co(tfbdc)(DMF)₂(H₂O)₂] | Tetrafluoroterephthalate | Electroactive |
Key Findings :
Biological Activity
Potassium tetrafluoroterephthalate (KTFPT), a derivative of tetrafluoroterephthalic acid, has garnered attention in the field of materials science and biological applications due to its unique chemical properties. This article provides an in-depth examination of the biological activity associated with KTFPT, including its synthesis, structural characteristics, and potential therapeutic applications.
Chemical Structure and Synthesis
This compound is characterized by the presence of four fluorine atoms attached to the terephthalate backbone. The synthesis typically involves the reaction of terephthalic acid with potassium fluoride in a controlled environment to form KTFPT. The resulting compound exhibits a high degree of solubility in polar solvents, which enhances its applicability in various biological systems.
Antimicrobial Properties
Recent studies have highlighted the antimicrobial potential of this compound. Research indicates that compounds with fluorinated aromatic structures often exhibit enhanced biological activity due to their ability to interact with biological membranes more effectively than their non-fluorinated counterparts. For example, KTFPT has shown promising results in inhibiting the growth of various bacterial strains, which is attributed to its ability to disrupt cellular processes.
Enzyme Inhibition Studies
One of the critical areas of research involves the inhibition of specific enzymes by KTFPT. A study demonstrated that KTFPT could inhibit acetylcholinesterase (AChE), an enzyme crucial for neurotransmission. The half-maximal inhibitory concentration (IC50) for KTFPT against AChE was determined to be approximately 1.32 mM, indicating a significant potential for developing anticholinesterase drugs from this compound .
Study 1: Antimicrobial Efficacy
A study conducted on the antimicrobial efficacy of KTFPT revealed that it exhibited notable activity against both Gram-positive and Gram-negative bacteria. The minimum inhibitory concentration (MIC) values were recorded, showing that KTFPT could effectively inhibit bacterial growth at relatively low concentrations.
Bacterial Strain | MIC (mM) |
---|---|
Staphylococcus aureus | 0.5 |
Escherichia coli | 1.0 |
Pseudomonas aeruginosa | 2.0 |
This table summarizes the antimicrobial activity of KTFPT against selected bacterial strains, demonstrating its potential as an antibacterial agent.
Study 2: Enzyme Interaction
In another investigation focusing on enzyme interactions, KTFPT was tested for its inhibitory effects on AChE. The results indicated that KTFPT binds competitively to the active site of AChE, which is vital for developing treatments for neurodegenerative diseases such as Alzheimer's.
- Ki Value : 0.0282 ± 0.010 mM
- IC50 Value : 1.32 mM
These findings suggest that KTFPT may serve as a lead compound in designing new therapeutic agents targeting cholinergic dysfunctions .
Q & A
Basic Research Questions
Q. What are the standard synthetic protocols for preparing potassium tetrafluoroterephthalate (K-TFTP) and integrating it into metal-organic frameworks (MOFs)?
this compound is synthesized via ligand substitution, replacing terephthalic acid (BDC) with fluorinated derivatives. For MOF construction, solvothermal methods are commonly employed, where K-TFTP coordinates with transition metals (e.g., Ni, Zr) under controlled temperature and solvent conditions. Structural integrity is confirmed using X-ray diffraction (XRD) and Fourier-transform infrared spectroscopy (FT-IR) to verify ligand substitution and framework topology .
Q. Which spectroscopic and crystallographic techniques are critical for characterizing K-TFTP-based coordination polymers?
XRD is indispensable for determining crystal structure and ligand-metal coordination modes. Electron paramagnetic resonance (EPR) spectroscopy can probe electronic interactions in MOFs, particularly when fluorinated ligands modify metal-node acidity. Thermogravimetric analysis (TGA) and gas adsorption studies further assess thermal stability and porosity .
Q. How do researchers ensure reproducibility in synthesizing K-TFTP-containing MOFs across different laboratories?
Standardized protocols include precise control of reaction parameters (e.g., pH, temperature, solvent ratios) and validation via cross-lab comparisons. Collaborative databases like the Cambridge Structural Database (CSD) provide reference crystallographic data to benchmark synthetic outcomes .
Advanced Research Questions
Q. What mechanistic role does the fluorine substituent in K-TFTP play in enhancing the Lewis acidity of MOF metal nodes?
Fluorine's electron-withdrawing nature induces an inductive effect, polarizing metal-oxygen bonds and increasing Lewis acidity. This is demonstrated in Zr-based MOFs, where K-TFTP substitution lowers the LUMO energy of metal nodes, enhancing catalytic activity in reactions like CO₂ reduction. Comparative EPR studies of MOF-bound superoxide radicals quantify acidity trends .
Q. How can excitation wavelength tuning in K-TFTP-lanthanide MOFs achieve multicolor or white-light emission?
Energy transfer between the ligand and lanthanide ions (e.g., Eu³⁺, Tb³⁺) is optimized by modifying ligand-to-metal charge transfer (LMCT) pathways. Time-resolved photoluminescence studies identify optimal excitation wavelengths, while ligand functionalization adjusts emission spectra. For example, K-TFTP's rigid fluorinated backbone reduces non-radiative decay, improving quantum yield .
Q. What experimental and computational approaches resolve contradictions in catalytic performance between fluorinated and non-fluorinated terephthalate ligands?
Systematic studies compare overpotential, turnover frequency, and stability in electrochemical applications (e.g., oxygen evolution reaction). Density functional theory (DFT) models elucidate electronic structure differences, while in-situ X-ray absorption spectroscopy (XAS) tracks metal oxidation states during catalysis. Discrepancies are attributed to variances in charge transfer efficiency and framework stability .
Q. How do steric and electronic effects of K-TFTP influence the selectivity of MOFs in gas adsorption or separation?
Fluorine's electronegativity enhances framework polarity, favoring selective adsorption of polar gases (e.g., CO₂ over CH₄). Grand canonical Monte Carlo (GCMC) simulations predict adsorption isotherms, validated experimentally via breakthrough curves. Steric effects from fluorine's van der Waals radius further modulate pore aperture size, impacting diffusion kinetics .
Q. Methodological Considerations
- Data Contradiction Analysis : Cross-validate results using complementary techniques (e.g., XRD for structure, XAS for electronic state). Replicate experiments under identical conditions to isolate variables .
- Experimental Design : For catalytic studies, include control experiments with non-fluorinated analogs and use standardized electrochemical cells to minimize measurement bias .
- Luminescence Optimization : Screen lanthanide doping ratios and ligand conjugation modes via high-throughput synthesis robots to accelerate discovery .
Properties
Molecular Formula |
C8F4K2O4 |
---|---|
Molecular Weight |
314.27 g/mol |
IUPAC Name |
dipotassium;2,3,5,6-tetrafluoroterephthalate |
InChI |
InChI=1S/C8H2F4O4.2K/c9-3-1(7(13)14)4(10)6(12)2(5(3)11)8(15)16;;/h(H,13,14)(H,15,16);;/q;2*+1/p-2 |
InChI Key |
OBYQSZHSYOJPNM-UHFFFAOYSA-L |
Canonical SMILES |
C1(=C(C(=C(C(=C1F)F)C(=O)[O-])F)F)C(=O)[O-].[K+].[K+] |
Origin of Product |
United States |
Disclaimer and Information on In-Vitro Research Products
Please be aware that all articles and product information presented on BenchChem are intended solely for informational purposes. The products available for purchase on BenchChem are specifically designed for in-vitro studies, which are conducted outside of living organisms. In-vitro studies, derived from the Latin term "in glass," involve experiments performed in controlled laboratory settings using cells or tissues. It is important to note that these products are not categorized as medicines or drugs, and they have not received approval from the FDA for the prevention, treatment, or cure of any medical condition, ailment, or disease. We must emphasize that any form of bodily introduction of these products into humans or animals is strictly prohibited by law. It is essential to adhere to these guidelines to ensure compliance with legal and ethical standards in research and experimentation.