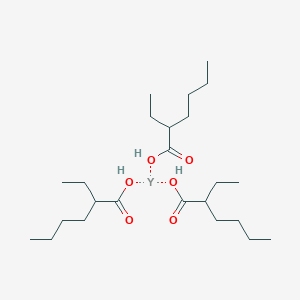
Tris(2-ethylhexanoyloxy)yttrium
- Click on QUICK INQUIRY to receive a quote from our team of experts.
- With the quality product at a COMPETITIVE price, you can focus more on your research.
Overview
Description
Tris(2-ethylhexanoyloxy)yttrium, also known as yttrium(III) 2-ethylhexanoate, is an organometallic compound with the molecular formula C₂₄H₄₅O₆Y and a molecular weight of 518.524 g/mol . It belongs to the class of yttrium carboxylates, where the yttrium(III) ion is coordinated by three 2-ethylhexanoate ligands. This compound is structurally characterized by bulky, branched alkyl chains that enhance its solubility in non-polar organic solvents.
Preparation Methods
Synthetic Routes and Reaction Conditions: Yttrium(III) 2-ethylhexanoate can be synthesized by reacting yttrium oxide (Y2O3) with 2-ethylhexanoic acid in the presence of a suitable solvent. The reaction typically occurs under reflux conditions, ensuring complete dissolution of yttrium oxide and formation of the desired product .
Industrial Production Methods: In industrial settings, the synthesis of yttrium(III) 2-ethylhexanoate often involves large-scale reactors where yttrium oxide is continuously fed into a reactor containing 2-ethylhexanoic acid. The reaction is maintained at elevated temperatures to ensure high yield and purity of the product .
Chemical Reactions Analysis
Types of Reactions: Yttrium(III) 2-ethylhexanoate undergoes various chemical reactions, including:
Oxidation: It can be oxidized to form yttrium(III) oxide.
Substitution: The 2-ethylhexanoate ligands can be substituted with other ligands, such as acetylacetonate or nitrate.
Common Reagents and Conditions:
Oxidation: Requires an oxidizing agent such as hydrogen peroxide or oxygen.
Substitution: Typically involves the use of a stronger ligand in a suitable solvent under reflux conditions.
Major Products:
Oxidation: Yttrium(III) oxide.
Substitution: Yttrium complexes with different ligands.
Scientific Research Applications
Materials Science
1.1. Thin Film Formation
Tris(2-ethylhexanoyloxy)yttrium plays a crucial role in the formation of thin films, particularly in the fabrication of ferroelectric materials. Its ability to form uniform films makes it an ideal candidate for applications in electronic devices. Studies have demonstrated that the compound can be used to create high-quality ferroelectric thin films through sol-gel processes, enhancing the performance of capacitors and memory devices .
1.2. Nanoparticle Synthesis
The compound is also utilized in synthesizing yttrium oxide nanoparticles, which are important for various applications, including catalysis and as phosphors in display technologies. Its coordination chemistry allows for the controlled growth of nanoparticles with specific sizes and morphologies, which can be tailored for particular applications .
Catalysis
2.1. Catalytic Properties
this compound exhibits catalytic properties that are beneficial in organic synthesis. It has been shown to facilitate reactions such as esterification and transesterification, providing a more efficient pathway for producing biodiesel from triglycerides .
2.2. Coordination Complexes
The compound's ability to form coordination complexes enhances its catalytic activity. For example, when combined with transition metal catalysts, it can significantly improve reaction rates and selectivity in various organic transformations .
Biomedical Applications
3.1. Drug Delivery Systems
Recent research highlights the potential of this compound in drug delivery systems due to its biocompatibility and ability to encapsulate therapeutic agents. Its structure allows for controlled release mechanisms, making it suitable for targeted therapy in cancer treatment.
3.2. Imaging Agents
The compound is being investigated as a contrast agent for imaging techniques such as MRI due to its paramagnetic properties when complexed with gadolinium or other MRI-active metals. This application could enhance imaging resolution and provide better diagnostic capabilities .
Case Studies
Mechanism of Action
The mechanism of action of yttrium(III) 2-ethylhexanoate involves the coordination of yttrium with the 2-ethylhexanoate ligands. This coordination stabilizes the yttrium ion and allows it to participate in various chemical reactions. The molecular targets and pathways depend on the specific application, such as catalysis or biomedical use .
Comparison with Similar Compounds
Comparison with Similar Yttrium Compounds
Structural and Physical Properties
The table below compares Tris(2-ethylhexanoyloxy)yttrium with structurally related yttrium complexes and salts:
Key Observations:
- Ligand Effects: The 2-ethylhexanoate ligand in this compound imparts greater hydrophobicity compared to simpler carboxylates like acetate. This enhances compatibility with organic matrices, making it suitable for homogeneous catalysis . In contrast, cyclopentadienyl ligands (e.g., in Tris(n-butylcyclopentadienyl)yttrium(III)) create highly reactive, air-sensitive complexes often used in vapor deposition or polymerization initiators .
- Stability: Cyclopentadienyl derivatives require inert handling due to flammability and reactivity with water/air , whereas yttrium carboxylates like this compound are likely more stable under ambient conditions.
- Solubility: Yttrium nitrate and acetate salts are water-soluble, enabling aqueous-phase applications, while this compound and cyclopentadienyl complexes are tailored for non-polar environments .
Biological Activity
Tris(2-ethylhexanoyloxy)yttrium (TEHY) is a compound of increasing interest in biomedical research due to its potential applications in drug delivery, imaging, and as a therapeutic agent. This article reviews the biological activity of TEHY, focusing on its cytotoxic effects, mechanisms of action, and potential therapeutic applications.
TEHY is a yttrium-based compound characterized by the presence of three 2-ethylhexanoyloxy groups. Its unique structure allows it to interact with biological systems in various ways, influencing cellular processes.
Table 1: Summary of Cytotoxic Effects Induced by Yttrium Compounds
Compound | Cell Type | Effect on Viability | Mechanism of Action |
---|---|---|---|
YCl₃ | Leydig cells | Decreased | Ca²⁺/IP3R1/CaMKII pathway |
TEHY (hypothetical) | Various | Unknown | Potentially similar to YCl₃ effects |
Study 1: Yttrium Chloride Induced Apoptosis
In a controlled study, rats exposed to YCl₃ demonstrated significant testicular damage characterized by increased apoptosis rates. The study highlighted the role of calcium signaling in mediating these effects, suggesting that similar yttrium compounds, including TEHY, may exhibit comparable cytotoxicity under specific conditions .
Study 2: Biocompatibility of Yttrium-Doped Materials
Research into yttrium-doped magnesium alloys indicated that the incorporation of yttrium can modulate degradation rates in biological environments. This suggests that yttrium compounds can influence cellular interactions and biocompatibility, which is critical for their application in biomedical devices . Although TEHY's specific interactions remain to be fully elucidated, its yttrium content may confer similar properties.
Potential Therapeutic Applications
The biological activity of TEHY suggests several potential applications:
- Drug Delivery Systems : Due to its ability to influence cell viability and apoptosis, TEHY could be engineered as a carrier for targeted drug delivery in cancer therapy.
- Imaging Agents : The unique optical properties associated with yttrium compounds may allow TEHY to be utilized in imaging techniques such as MRI or fluorescence imaging.
- Bone Regeneration : Yttrium's role in modulating degradation rates in magnesium alloys points towards potential applications in bone repair materials.
Q & A
Basic Research Questions
Q. What synthetic methodologies are reported for Tris(2-ethylhexanoyloxy)yttrium, and how do reaction parameters influence product yield and purity?
- Methodological Answer : Synthesis typically involves ligand exchange reactions under inert atmospheres to prevent hydrolysis or oxidation. For analogous yttrium complexes (e.g., Tris(n-butylcyclopentadienyl)yttrium(III)), protocols recommend using anhydrous solvents like tetrahydrofuran and maintaining temperatures between 0–25°C to optimize ligand coordination . Variations in stoichiometry, solvent polarity, and reaction duration should be systematically tested to identify optimal conditions.
Q. Which spectroscopic techniques are most effective for characterizing the coordination geometry and purity of this compound?
- Methodological Answer : Nuclear Magnetic Resonance (NMR) spectroscopy (¹H, ¹³C, and ⁸⁹Y) is critical for verifying ligand attachment and metal-ligand bonding. For example, ⁸⁹Y NMR can resolve yttrium coordination environments in related complexes . Complementary techniques like Fourier-Transform Infrared (FTIR) spectroscopy and X-ray crystallography should be employed to confirm ligand binding modes and crystallinity .
Q. What are the recommended storage conditions to prevent degradation of this compound in laboratory settings?
- Methodological Answer : Storage under inert gas (argon or nitrogen) in sealed, moisture-resistant containers is essential, as yttrium organometallics are often hygroscopic and reactive toward atmospheric moisture. Stability studies for analogous compounds suggest refrigeration (0–6°C) further extends shelf life .
Advanced Research Questions
Q. How can researchers resolve discrepancies in reported thermal stability data for this compound under varying atmospheric conditions?
- Methodological Answer : Contradictory stability data may arise from differences in experimental setups (e.g., open vs. closed systems, humidity levels). Controlled thermogravimetric analysis (TGA) under inert vs. ambient atmospheres can clarify decomposition pathways. For example, Tris(n-butylcyclopentadienyl)yttrium(III) decomposes at 120°C in air but remains stable up to 200°C under argon . Parallel experiments with standardized protocols are recommended to isolate variables.
Q. What strategies mitigate ligand dissociation during catalytic applications of this compound?
- Methodological Answer : Ligand dissociation can be minimized by using sterically bulky counter-ligands or optimizing reaction solvents to stabilize the yttrium center. For instance, coordinating solvents like dimethylformamide (DMF) may reduce dissociation rates. Kinetic studies comparing ligand exchange rates in polar vs. nonpolar solvents could identify stabilization mechanisms .
Q. How does the choice of ancillary ligands impact the reactivity of this compound in polymerization or catalysis?
- Methodological Answer : Systematic ligand substitution studies (e.g., replacing 2-ethylhexanoyloxy with cyclopentadienyl or acetylacetonate groups) can reveal steric/electronic effects on reactivity. For example, bulky ligands in Tris(n-propylcyclopentadienyl)yttrium(III) enhance thermal stability but reduce catalytic activity in olefin polymerization . Density Functional Theory (DFT) calculations may further elucidate ligand-metal interactions.
Q. Data Analysis and Experimental Design
Q. What statistical approaches are suitable for analyzing reproducibility issues in the synthesis of this compound?
- Methodological Answer : Design-of-Experiments (DoE) frameworks, such as factorial designs, can identify critical variables (e.g., temperature, solvent purity) affecting yield and reproducibility. Multivariate analysis of variance (ANOVA) helps quantify the significance of each parameter .
Q. How should researchers address conflicting reports on the hydrolytic stability of this compound in aqueous-organic biphasic systems?
- Methodological Answer : Controlled hydrolysis experiments with pH monitoring and kinetic profiling can resolve contradictions. For example, in analogous yttrium nitrate hexahydrate systems, hydrolysis rates increase exponentially above pH 5.0 . In-situ techniques like Raman spectroscopy may track ligand displacement dynamics .
Q. Safety and Environmental Considerations
Q. What safety protocols are critical when handling this compound in open-air laboratories?
- Methodological Answer : Use gloveboxes or Schlenk lines for air-sensitive manipulations. Personal protective equipment (PPE) must include nitrile gloves, flame-resistant lab coats, and face shields. Emergency protocols for fires should specify dry-powder extinguishers, as water-reactive yttrium compounds may release flammable gases .
Q. How can researchers assess the environmental impact of this compound waste in academic labs?
Properties
Molecular Formula |
C24H48O6Y |
---|---|
Molecular Weight |
521.5 g/mol |
IUPAC Name |
2-ethylhexanoic acid;yttrium |
InChI |
InChI=1S/3C8H16O2.Y/c3*1-3-5-6-7(4-2)8(9)10;/h3*7H,3-6H2,1-2H3,(H,9,10); |
InChI Key |
DWQHHFSPGQRLMB-UHFFFAOYSA-N |
Canonical SMILES |
CCCCC(CC)C(=O)O.CCCCC(CC)C(=O)O.CCCCC(CC)C(=O)O.[Y] |
Origin of Product |
United States |
Disclaimer and Information on In-Vitro Research Products
Please be aware that all articles and product information presented on BenchChem are intended solely for informational purposes. The products available for purchase on BenchChem are specifically designed for in-vitro studies, which are conducted outside of living organisms. In-vitro studies, derived from the Latin term "in glass," involve experiments performed in controlled laboratory settings using cells or tissues. It is important to note that these products are not categorized as medicines or drugs, and they have not received approval from the FDA for the prevention, treatment, or cure of any medical condition, ailment, or disease. We must emphasize that any form of bodily introduction of these products into humans or animals is strictly prohibited by law. It is essential to adhere to these guidelines to ensure compliance with legal and ethical standards in research and experimentation.