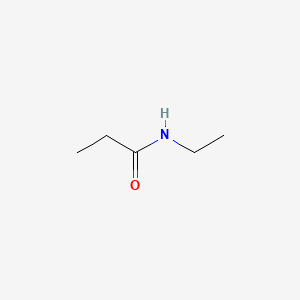
N-Ethylpropionamide
Overview
Description
N-Ethylpropionamide is an organic compound with the molecular formula C5H11NO. It belongs to the class of amides, which are characterized by the presence of a carbonyl group (C=O) bonded to a nitrogen atom.
Preparation Methods
Synthetic Routes and Reaction Conditions: N-Ethylpropionamide can be synthesized through the reaction of propionyl chloride with ethylamine. The reaction typically occurs in the presence of a base such as pyridine to neutralize the hydrochloric acid formed during the process. The reaction can be represented as follows:
CH3CH2COCl+C2H5NH2→CH3CH2CONHC2H5+HCl
Industrial Production Methods: In industrial settings, this compound can be produced using similar methods but on a larger scale. The reaction conditions are optimized to ensure high yield and purity of the product. The use of continuous flow reactors and automated systems can enhance the efficiency of the production process .
Chemical Reactions Analysis
Types of Reactions: N-Ethylpropionamide undergoes various chemical reactions, including:
Hydrolysis: In the presence of strong acids or bases, this compound can be hydrolyzed to produce propionic acid and ethylamine.
Oxidation: Oxidizing agents can convert this compound to corresponding carboxylic acids.
Substitution: The amide group can participate in nucleophilic substitution reactions, where the nitrogen atom is replaced by other nucleophiles.
Common Reagents and Conditions:
Hydrolysis: Strong acids (e.g., hydrochloric acid) or bases (e.g., sodium hydroxide) under reflux conditions.
Oxidation: Oxidizing agents such as potassium permanganate or chromium trioxide.
Substitution: Nucleophiles such as amines or alcohols in the presence of catalysts.
Major Products:
Hydrolysis: Propionic acid and ethylamine.
Oxidation: Propionic acid.
Substitution: Various substituted amides depending on the nucleophile used.
Scientific Research Applications
N-Ethylpropionamide has several applications in scientific research:
Chemistry: Used as a building block in organic synthesis and as a reagent in various chemical reactions.
Biology: Studied for its potential biological activities and interactions with biomolecules.
Medicine: Investigated for its potential therapeutic properties and as a precursor for drug development.
Industry: Utilized in the production of polymers, resins, and other industrial chemicals.
Mechanism of Action
The mechanism of action of N-Ethylpropionamide involves its interaction with various molecular targets. The amide group can form hydrogen bonds with other molecules, influencing their structure and function. In biological systems, this compound can interact with enzymes and proteins, affecting their activity and stability. The exact pathways and molecular targets depend on the specific application and context of use .
Comparison with Similar Compounds
N-Methylpropionamide: Similar structure but with a methyl group instead of an ethyl group.
N-Propylpropionamide: Similar structure but with a propyl group instead of an ethyl group.
N-Ethylacetamide: Similar structure but with an acetamide group instead of a propionamide group.
Uniqueness: N-Ethylpropionamide is unique due to its specific combination of an ethyl group and a propionamide group. This combination imparts distinct chemical and physical properties, making it suitable for specific applications where other similar compounds may not be as effective .
Properties
IUPAC Name |
N-ethylpropanamide | |
---|---|---|
Source | PubChem | |
URL | https://pubchem.ncbi.nlm.nih.gov | |
Description | Data deposited in or computed by PubChem | |
InChI |
InChI=1S/C5H11NO/c1-3-5(7)6-4-2/h3-4H2,1-2H3,(H,6,7) | |
Source | PubChem | |
URL | https://pubchem.ncbi.nlm.nih.gov | |
Description | Data deposited in or computed by PubChem | |
InChI Key |
ABMDIECEEGFXNC-UHFFFAOYSA-N | |
Source | PubChem | |
URL | https://pubchem.ncbi.nlm.nih.gov | |
Description | Data deposited in or computed by PubChem | |
Canonical SMILES |
CCC(=O)NCC | |
Source | PubChem | |
URL | https://pubchem.ncbi.nlm.nih.gov | |
Description | Data deposited in or computed by PubChem | |
Molecular Formula |
C5H11NO | |
Source | PubChem | |
URL | https://pubchem.ncbi.nlm.nih.gov | |
Description | Data deposited in or computed by PubChem | |
DSSTOX Substance ID |
DTXSID40334619 | |
Record name | N-Ethylpropionamide | |
Source | EPA DSSTox | |
URL | https://comptox.epa.gov/dashboard/DTXSID40334619 | |
Description | DSSTox provides a high quality public chemistry resource for supporting improved predictive toxicology. | |
Molecular Weight |
101.15 g/mol | |
Source | PubChem | |
URL | https://pubchem.ncbi.nlm.nih.gov | |
Description | Data deposited in or computed by PubChem | |
CAS No. |
5129-72-6 | |
Record name | N-Ethylpropionamide | |
Source | EPA DSSTox | |
URL | https://comptox.epa.gov/dashboard/DTXSID40334619 | |
Description | DSSTox provides a high quality public chemistry resource for supporting improved predictive toxicology. | |
Retrosynthesis Analysis
AI-Powered Synthesis Planning: Our tool employs the Template_relevance Pistachio, Template_relevance Bkms_metabolic, Template_relevance Pistachio_ringbreaker, Template_relevance Reaxys, Template_relevance Reaxys_biocatalysis model, leveraging a vast database of chemical reactions to predict feasible synthetic routes.
One-Step Synthesis Focus: Specifically designed for one-step synthesis, it provides concise and direct routes for your target compounds, streamlining the synthesis process.
Accurate Predictions: Utilizing the extensive PISTACHIO, BKMS_METABOLIC, PISTACHIO_RINGBREAKER, REAXYS, REAXYS_BIOCATALYSIS database, our tool offers high-accuracy predictions, reflecting the latest in chemical research and data.
Strategy Settings
Precursor scoring | Relevance Heuristic |
---|---|
Min. plausibility | 0.01 |
Model | Template_relevance |
Template Set | Pistachio/Bkms_metabolic/Pistachio_ringbreaker/Reaxys/Reaxys_biocatalysis |
Top-N result to add to graph | 6 |
Feasible Synthetic Routes
Q1: Why is N-Ethylpropionamide useful for studying peptides?
A1: NEPA serves as a simplified model for studying the structural dynamics and interactions of β-peptides. [, , ] Its amide-I vibrational characteristics, particularly the N-H stretching vibration (amide-A mode), are sensitive to molecular structure, chemical environment, and hydrogen bonding, mirroring similar behaviors observed in larger peptides. [, , , ]
Q2: How do different solvents affect the behavior of this compound?
A2: Research shows that NEPA exhibits different aggregation states depending on the solvent. [] In polar solvents like DMSO and acetonitrile, NEPA favors monomeric and dimeric forms, while in nonpolar solvents such as chloroform and carbon tetrachloride, oligomers become more prevalent. [] This difference arises from the varying strengths of hydrogen bonding interactions between NEPA and the solvent molecules.
Q3: What insights have been gained about this compound using two-dimensional infrared (2D IR) spectroscopy?
A3: 2D IR spectroscopy has proven invaluable in understanding NEPA's ultrafast structural dynamics. Experiments revealed a spectral diffusion time of approximately 1 picosecond, suggesting a similar timescale for the structural dynamics of hydrogen bonding between NEPA's amide group and surrounding water molecules. [, ] This technique also allowed researchers to selectively probe specific NEPA aggregates, like dimers and trimers, revealing differences in their vibrational lifetimes and anharmonicities. []
Q4: Can we predict the vibrational frequencies of this compound in different environments?
A4: Researchers have developed a general applicable amide-I vibrational frequency map (GA map) for β-peptides using NEPA as a model. [] This map utilizes a combination of ab initio and molecular mechanics calculations to accurately describe molecular structures and solute-solvent interactions, enabling researchers to predict the amide-I vibrational frequencies of NEPA and other β-peptides in various solvents. []
Q5: How does this compound interact with metal ions in solution?
A5: Studies using infrared spectroscopy and molecular dynamics simulations showed that the interaction of NEPA with metal cations like Na+, Ca2+, Mg2+, and Al3+ is mediated by water molecules. [] The presence of these cations perturbs the hydrogen bonding network around NEPA, leading to a split in the amide-I absorption band. [] This split signifies the presence of both salting-in and salting-out effects on the NEPA molecule due to dynamic interactions with the surrounding water and ions. []
Q6: Are there any enzymatic activities related to this compound?
A6: While NEPA itself might not be a natural substrate, research has identified an inducible amidase enzyme in Pseudomonas aeruginosa that can hydrolyze similar compounds. [, ] This enzyme exhibits activity towards amides like acetamide, propionamide, and even some N-alkyl-substituted amides. [, ] Interestingly, the enzyme's activity is affected by the size and structure of the amide substrate. [, ]
Disclaimer and Information on In-Vitro Research Products
Please be aware that all articles and product information presented on BenchChem are intended solely for informational purposes. The products available for purchase on BenchChem are specifically designed for in-vitro studies, which are conducted outside of living organisms. In-vitro studies, derived from the Latin term "in glass," involve experiments performed in controlled laboratory settings using cells or tissues. It is important to note that these products are not categorized as medicines or drugs, and they have not received approval from the FDA for the prevention, treatment, or cure of any medical condition, ailment, or disease. We must emphasize that any form of bodily introduction of these products into humans or animals is strictly prohibited by law. It is essential to adhere to these guidelines to ensure compliance with legal and ethical standards in research and experimentation.