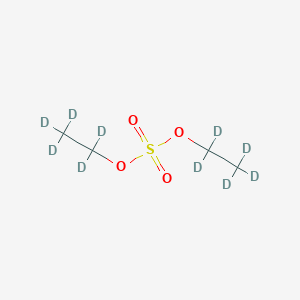
Diethyl sulfate-d10
- Click on QUICK INQUIRY to receive a quote from our team of experts.
- With the quality product at a COMPETITIVE price, you can focus more on your research.
Overview
Description
Diethyl sulfate-d10 is a deuterated form of diethyl sulfate, an organosulfur compound with the formula (C2D5O)2SO2. It is a colorless, oily liquid that is toxic, combustible, and likely carcinogenic. This compound is used primarily as an ethylating agent in various chemical reactions.
Preparation Methods
Synthetic Routes and Reaction Conditions
Diethyl sulfate-d10 can be synthesized through a two-step process starting from chlorosulfuric acid and deuterated ethanol (C2D5OH). The reaction proceeds as follows:
- Chlorosulfuric acid reacts with deuterated ethanol to form deuterated ethyl sulfate and hydrogen chloride:
ClSO3H+C2D5OH→C2D5OSO3H+HCl
- The resulting deuterated ethyl sulfate is then heated with sodium sulfate, leading to a redistribution reaction:
2C2D5OSO3H+Na2SO4→(C2D5O)2SO2+2NaHSO4
Industrial Production Methods
Industrial production of this compound follows similar synthetic routes but on a larger scale. The reaction conditions are optimized to ensure high yield and purity of the final product. The use of deuterated ethanol ensures the incorporation of deuterium atoms into the compound.
Chemical Reactions Analysis
Types of Reactions
Diethyl sulfate-d10 undergoes various types of chemical reactions, including:
Substitution Reactions: It acts as an ethylating agent, transferring ethyl groups to nucleophiles.
Hydrolysis: It hydrolyzes readily in the presence of water, forming deuterated ethanol and deuterated ethyl sulfate.
Common Reagents and Conditions
Nucleophiles: this compound reacts with nucleophiles such as sodium phenoxide, potassium iodide, and sodium acetate.
Conditions: Reactions typically occur under mild conditions, often at room temperature or slightly elevated temperatures.
Major Products
Ethyl Ethers: Reaction with phenoxides forms ethyl ethers.
Ethyl Iodide: Reaction with potassium iodide forms ethyl iodide.
Ethyl Esters: Reaction with carboxylates forms ethyl esters.
Scientific Research Applications
Diethyl sulfate-d10 is used in various scientific research applications, including:
Chemistry: As an ethylating agent, it is used in the synthesis of ethylated compounds.
Biology: It is used in mutagenesis studies to induce mutations in genetic material.
Medicine: It is used in the development of pharmaceuticals and other therapeutic agents.
Industry: It is used in the production of dyes, perfumes, and other industrial chemicals.
Mechanism of Action
Diethyl sulfate-d10 exerts its effects through alkylation, transferring ethyl groups to nucleophilic sites on molecules. This process involves the formation of a positively charged ethyl group that reacts with nucleophiles, leading to the formation of ethylated products. The molecular targets include nucleophilic sites on DNA, proteins, and other biomolecules, which can result in mutations and other chemical modifications.
Comparison with Similar Compounds
Similar Compounds
Dimethyl Sulfate: Another alkylating agent with similar properties but uses methyl groups instead of ethyl groups.
Diethyl Sulfite: Similar in structure but has different reactivity and applications.
Uniqueness
Diethyl sulfate-d10 is unique due to the presence of deuterium atoms, which can be useful in isotopic labeling studies. The deuterium atoms provide a distinct mass difference, making it easier to track and analyze the compound in various chemical and biological systems.
Properties
CAS No. |
96617-81-1 |
---|---|
Molecular Formula |
C4H10O4S |
Molecular Weight |
164.25 g/mol |
IUPAC Name |
bis(1,1,2,2,2-pentadeuterioethyl) sulfate |
InChI |
InChI=1S/C4H10O4S/c1-3-7-9(5,6)8-4-2/h3-4H2,1-2H3/i1D3,2D3,3D2,4D2 |
InChI Key |
DENRZWYUOJLTMF-MWUKXHIBSA-N |
Isomeric SMILES |
[2H]C([2H])([2H])C([2H])([2H])OS(=O)(=O)OC([2H])([2H])C([2H])([2H])[2H] |
Canonical SMILES |
CCOS(=O)(=O)OCC |
Origin of Product |
United States |
Disclaimer and Information on In-Vitro Research Products
Please be aware that all articles and product information presented on BenchChem are intended solely for informational purposes. The products available for purchase on BenchChem are specifically designed for in-vitro studies, which are conducted outside of living organisms. In-vitro studies, derived from the Latin term "in glass," involve experiments performed in controlled laboratory settings using cells or tissues. It is important to note that these products are not categorized as medicines or drugs, and they have not received approval from the FDA for the prevention, treatment, or cure of any medical condition, ailment, or disease. We must emphasize that any form of bodily introduction of these products into humans or animals is strictly prohibited by law. It is essential to adhere to these guidelines to ensure compliance with legal and ethical standards in research and experimentation.