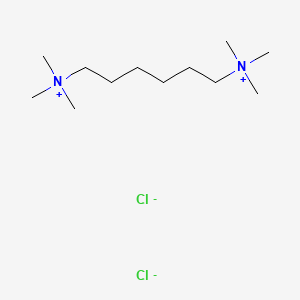
Hexamethonium chloride
Overview
Description
Hexamethonium chloride is an organic compound with the chemical formula C12H30Cl2N2 . It is a quaternary ammonium compound that is soluble in water and ethanol but nearly insoluble in chloroform and ether . This compound is primarily known for its role as a ganglionic blocker, which means it inhibits transmission in autonomic ganglia by blocking nicotinic acetylcholine receptors .
Mechanism of Action
Target of Action
Hexamethonium chloride primarily targets the neuronal nicotinic acetylcholine receptors (nAChR) . These receptors play a crucial role in transmitting signals in autonomic ganglia, which are clusters of nerve cells that relay information to the peripheral nervous system .
Mode of Action
This compound acts as a non-depolarising ganglionic blocker . This interaction primarily blocks the ion pore of the neuronal nicotinic receptors, rather than competing with the binding site for acetylcholine . This blockage inhibits both the sympathetic and parasympathetic nervous systems .
Biochemical Pathways
It is known that by blocking the neuronal nicotinic receptors in autonomic ganglia, this compound inhibits the transmission in all autonomic ganglia, affecting both the sympathetic and parasympathetic nervous systems .
Result of Action
The blockage of neuronal nicotinic receptors by this compound results in the inhibition of both the sympathetic and parasympathetic nervous systems . This leads to various physiological effects, including orthostatic hypotension and sexual dysfunction (sympatholytic effects), and constipation, urinary retention, glaucoma, blurry vision, decreased lacrimal gland secretion, and dry mouth (parasympatholytic effects) .
Action Environment
The action, efficacy, and stability of this compound can be influenced by various environmental factors.
Biochemical Analysis
Biochemical Properties
Hexamethonium chloride functions primarily by blocking neuronal nicotinic acetylcholine receptors (nAChRs) in autonomic ganglia. This blockade inhibits the transmission of nerve impulses in both the sympathetic and parasympathetic nervous systems. The compound does not affect muscarinic acetylcholine receptors (mAChRs) or nicotinic receptors at the skeletal neuromuscular junction . This compound interacts with the ion pore of nAChRs, preventing the flow of ions and thus inhibiting neuronal signaling .
Cellular Effects
This compound exerts significant effects on various cell types by inhibiting the function of nAChRs. This inhibition disrupts normal cell signaling pathways, leading to altered cellular functions. In autonomic ganglia, the blockade of nAChRs by this compound results in the inhibition of neurotransmitter release, affecting processes such as heart rate, blood pressure, and gastrointestinal motility . Additionally, this compound can influence gene expression and cellular metabolism by altering intracellular signaling cascades .
Molecular Mechanism
At the molecular level, this compound acts by binding to the ion pore of nAChRs, blocking the passage of ions such as sodium and potassium. This blockade prevents the depolarization of the neuronal membrane, thereby inhibiting the transmission of nerve impulses . This compound does not compete with acetylcholine for the binding site but rather obstructs the ion channel itself . This mechanism of action leads to the inhibition of both sympathetic and parasympathetic nervous system activity .
Temporal Effects in Laboratory Settings
In laboratory settings, the effects of this compound can vary over time. The compound is relatively stable but may degrade under certain conditions. Long-term exposure to this compound in in vitro studies has shown sustained inhibition of nAChRs, leading to prolonged suppression of neuronal activity . In in vivo studies, the effects of this compound may diminish over time due to metabolic degradation and clearance from the body .
Dosage Effects in Animal Models
The effects of this compound in animal models are dose-dependent. At low doses, this compound effectively blocks nAChRs, leading to reduced autonomic nervous system activity . At higher doses, the compound can cause significant adverse effects, including hypotension, bradycardia, and gastrointestinal disturbances . Toxicity studies have shown that excessive doses of this compound can lead to severe autonomic dysfunction and even death in animal models .
Metabolic Pathways
This compound is poorly absorbed from the gastrointestinal tract and does not cross the blood-brain barrier . The compound is primarily metabolized in the liver, where it undergoes biotransformation to inactive metabolites . These metabolites are then excreted via the kidneys . The metabolic pathways of this compound involve various liver enzymes, including cytochrome P450 monooxygenases .
Transport and Distribution
This compound is distributed throughout the body via the bloodstream but does not readily cross the blood-brain barrier . The compound is transported to various tissues, including the autonomic ganglia, where it exerts its pharmacological effects . This compound is also known to interact with specific transporters and binding proteins that facilitate its distribution within cells .
Subcellular Localization
Within cells, this compound is localized primarily to the plasma membrane, where it interacts with nAChRs . The compound does not appear to accumulate in other subcellular compartments or organelles . The localization of this compound to the plasma membrane is crucial for its function as a ganglionic blocker, as it allows the compound to effectively inhibit nAChRs and disrupt neuronal signaling .
Preparation Methods
Hexamethonium chloride can be synthesized through the alkylation of 1,6-dichlorohexane with trimethylamine in methanol or ethanol under heating conditions . The reaction typically proceeds for 24 hours to ensure complete conversion. Industrial production methods may involve similar synthetic routes but on a larger scale, with optimized reaction conditions to maximize yield and purity.
Chemical Reactions Analysis
Hexamethonium chloride undergoes several types of chemical reactions:
Substitution Reactions: It can participate in nucleophilic substitution reactions due to the presence of chloride ions.
Oxidation and Reduction: While specific oxidation and reduction reactions are less common, the compound’s quaternary ammonium structure can influence its reactivity under certain conditions.
Common Reagents and Conditions: Typical reagents include for substitution reactions, and reactions are often carried out in polar solvents like water or ethanol. Major products depend on the specific reagents and conditions used but generally involve the replacement of chloride ions with other nucleophiles.
Scientific Research Applications
Hexamethonium chloride has a wide range of applications in scientific research:
Chemistry: It is used as a reagent in organic synthesis and as a standard compound in analytical chemistry.
Biology: The compound is employed to study the function of nicotinic acetylcholine receptors in autonomic ganglia.
Comparison with Similar Compounds
Hexamethonium chloride is unique among ganglionic blockers due to its specific structure and mechanism of action. Similar compounds include:
Decamethonium: Another ganglionic blocker but with a different structure and slightly different pharmacological profile.
Phenacyl Homatropinium Chloride: A quaternary ammonium compound with ganglionic blocking activity but a shorter duration of action.
This compound stands out due to its specific binding to nicotinic acetylcholine receptors and its historical significance in the treatment of hypertension.
Properties
CAS No. |
60-25-3 |
---|---|
Molecular Formula |
C12H30ClN2+ |
Molecular Weight |
237.83 g/mol |
IUPAC Name |
trimethyl-[6-(trimethylazaniumyl)hexyl]azanium;chloride |
InChI |
InChI=1S/C12H30N2.ClH/c1-13(2,3)11-9-7-8-10-12-14(4,5)6;/h7-12H2,1-6H3;1H/q+2;/p-1 |
InChI Key |
PDHQYSISHJHEKV-UHFFFAOYSA-M |
SMILES |
C[N+](C)(C)CCCCCC[N+](C)(C)C.[Cl-].[Cl-] |
Canonical SMILES |
C[N+](C)(C)CCCCCC[N+](C)(C)C.[Cl-] |
60-25-3 | |
physical_description |
Other Solid; Liquid |
Related CAS |
60-26-4 (Parent) |
Synonyms |
Bitartrate, Hexamethonium Bromide, Hexamethonium Chloride, Hexamethonium Depressin Dibromide Dihydrate, Hexamethonium Dibromide, Hexamethonium Dichloride Dihydrate, Hexamethonium Dihydrate, Hexamethonium Dibromide Dihydrate, Hexamethonium Dichloride Dihydroxide, Hexamethonium Diiodide, Hexamethonium Dimethylsulfate, Hexamethonium Diperchlorate, Hexamethonium Hexamethonium Hexamethonium Bitartrate Hexamethonium Bromide Hexamethonium Chloride Hexamethonium Dibromide Hexamethonium Dibromide Dihydrate Hexamethonium Dichloride Dihydrate Hexamethonium Dihydroxide Hexamethonium Diiodide Hexamethonium Dimethylsulfate Hexamethonium Diperchlorate Hexamethonium Iodide Hexamethonium Monotartrate Hexonium Iodide, Hexamethonium Monotartrate, Hexamethonium |
Origin of Product |
United States |
Retrosynthesis Analysis
AI-Powered Synthesis Planning: Our tool employs the Template_relevance Pistachio, Template_relevance Bkms_metabolic, Template_relevance Pistachio_ringbreaker, Template_relevance Reaxys, Template_relevance Reaxys_biocatalysis model, leveraging a vast database of chemical reactions to predict feasible synthetic routes.
One-Step Synthesis Focus: Specifically designed for one-step synthesis, it provides concise and direct routes for your target compounds, streamlining the synthesis process.
Accurate Predictions: Utilizing the extensive PISTACHIO, BKMS_METABOLIC, PISTACHIO_RINGBREAKER, REAXYS, REAXYS_BIOCATALYSIS database, our tool offers high-accuracy predictions, reflecting the latest in chemical research and data.
Strategy Settings
Precursor scoring | Relevance Heuristic |
---|---|
Min. plausibility | 0.01 |
Model | Template_relevance |
Template Set | Pistachio/Bkms_metabolic/Pistachio_ringbreaker/Reaxys/Reaxys_biocatalysis |
Top-N result to add to graph | 6 |
Feasible Synthetic Routes
Disclaimer and Information on In-Vitro Research Products
Please be aware that all articles and product information presented on BenchChem are intended solely for informational purposes. The products available for purchase on BenchChem are specifically designed for in-vitro studies, which are conducted outside of living organisms. In-vitro studies, derived from the Latin term "in glass," involve experiments performed in controlled laboratory settings using cells or tissues. It is important to note that these products are not categorized as medicines or drugs, and they have not received approval from the FDA for the prevention, treatment, or cure of any medical condition, ailment, or disease. We must emphasize that any form of bodily introduction of these products into humans or animals is strictly prohibited by law. It is essential to adhere to these guidelines to ensure compliance with legal and ethical standards in research and experimentation.