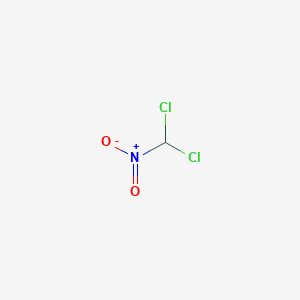
Dichloronitromethane
Overview
Description
Dichloronitromethane is a colorless liquid with an unpleasant odor . It is one of the Halonitromethanes, which are a recently identified class of disinfection by-products (DBPs) in drinking water .
Molecular Structure Analysis
The molecular formula of this compound is CHCl₂NO₂ . Its molecular weight is 129.930 Da .Physical and Chemical Properties Analysis
This compound has a density of 1.6±0.1 g/cm³, a boiling point of 107.0±0.0 °C at 760 mmHg, and a flash point of 23.2±21.8 °C . It has a vapor pressure of 27.6±0.2 mmHg at 25°C .Scientific Research Applications
Environmental Impact and Water Treatment
- Drinking Water Disinfection Byproducts : Dichloronitromethane is identified as a halonitromethane drinking water disinfection byproduct. Studies have shown it to be a potent mammalian cell cytotoxin and genotoxin, suggesting a hazard to public health and the environment (Plewa et al., 2004).
- Degradation Processes : Research on advanced reduction processes (ARPs) indicates successful degradation of harmful chemicals like 1,2-dichloroethane, hinting at potential applications for removing similar contaminants, including DCNM, from water (Liu et al., 2014).
- UV Photolysis for Removal : Studies on the pH-dependent degradation kinetics and pathways of halonitromethanes, including DCNM, by UV photolysis, offer insights into effective removal strategies from drinking water, highlighting the importance of adjusting pH levels for enhanced degradation rates (Fang et al., 2013).
Health and Environmental Risks
- Cytotoxicity and Genotoxicity : Research on halonitromethanes, including DCNM, emphasizes their cytotoxic and genotoxic properties, underlining the need for evaluating their impact on human health and potential risks associated with their presence in drinking water (Kundu et al., 2004).
- Degradation Mechanisms and Pathways : Investigations into the degradation mechanisms of halonitromethanes through advanced oxidation and reduction processes shed light on potential approaches to mitigate their presence and reduce their harmful effects (Mincher et al., 2010).
Analytical Techniques and Monitoring
- Detection and Quantification : Development of analytical methods, such as cold on-column injection coupled with gas chromatography/mass spectrometry, facilitates the accurate detection and quantification of halonitromethanes, including DCNM, in water sources. This is critical for monitoring water quality and assessing the effectiveness of water treatment processes (Chen et al., 2016).
Safety and Hazards
Mechanism of Action
Dichloronitromethane, also known as Methane, dichloronitro-, is a chemical compound with the formula CHCl₂NO₂ . It is a synthetic substance and is considered a potential groundwater pollutant .
Target of Action
It is known to be a metabolite of chloropicrin in soil and groundwater .
Mode of Action
It is a chemical transformation product
Biochemical Pathways
As a metabolite of chloropicrin, it may be involved in the biochemical pathways related to chloropicrin’s action .
Pharmacokinetics
It is known to have a potential for particle-bound transport .
Result of Action
It is known to be a possible groundwater pollutant , indicating that it may have environmental impacts.
Action Environment
Environmental factors can influence the action, efficacy, and stability of this compound. For instance, its potential for particle-bound transport suggests that it may be influenced by the presence of particles in the environment . .
Properties
IUPAC Name |
dichloro(nitro)methane | |
---|---|---|
Source | PubChem | |
URL | https://pubchem.ncbi.nlm.nih.gov | |
Description | Data deposited in or computed by PubChem | |
InChI |
InChI=1S/CHCl2NO2/c2-1(3)4(5)6/h1H | |
Source | PubChem | |
URL | https://pubchem.ncbi.nlm.nih.gov | |
Description | Data deposited in or computed by PubChem | |
InChI Key |
XUNYLLBGLKGFHO-UHFFFAOYSA-N | |
Source | PubChem | |
URL | https://pubchem.ncbi.nlm.nih.gov | |
Description | Data deposited in or computed by PubChem | |
Canonical SMILES |
C([N+](=O)[O-])(Cl)Cl | |
Source | PubChem | |
URL | https://pubchem.ncbi.nlm.nih.gov | |
Description | Data deposited in or computed by PubChem | |
Molecular Formula |
CHCl2NO2 | |
Source | PubChem | |
URL | https://pubchem.ncbi.nlm.nih.gov | |
Description | Data deposited in or computed by PubChem | |
DSSTOX Substance ID |
DTXSID30221387 | |
Record name | Methane, dichloronitro- | |
Source | EPA DSSTox | |
URL | https://comptox.epa.gov/dashboard/DTXSID30221387 | |
Description | DSSTox provides a high quality public chemistry resource for supporting improved predictive toxicology. | |
Molecular Weight |
129.93 g/mol | |
Source | PubChem | |
URL | https://pubchem.ncbi.nlm.nih.gov | |
Description | Data deposited in or computed by PubChem | |
CAS No. |
7119-89-3 | |
Record name | Dichloronitromethane | |
Source | CAS Common Chemistry | |
URL | https://commonchemistry.cas.org/detail?cas_rn=7119-89-3 | |
Description | CAS Common Chemistry is an open community resource for accessing chemical information. Nearly 500,000 chemical substances from CAS REGISTRY cover areas of community interest, including common and frequently regulated chemicals, and those relevant to high school and undergraduate chemistry classes. This chemical information, curated by our expert scientists, is provided in alignment with our mission as a division of the American Chemical Society. | |
Explanation | The data from CAS Common Chemistry is provided under a CC-BY-NC 4.0 license, unless otherwise stated. | |
Record name | Methane, dichloronitro- | |
Source | ChemIDplus | |
URL | https://pubchem.ncbi.nlm.nih.gov/substance/?source=chemidplus&sourceid=0007119893 | |
Description | ChemIDplus is a free, web search system that provides access to the structure and nomenclature authority files used for the identification of chemical substances cited in National Library of Medicine (NLM) databases, including the TOXNET system. | |
Record name | Methane, dichloronitro- | |
Source | EPA DSSTox | |
URL | https://comptox.epa.gov/dashboard/DTXSID30221387 | |
Description | DSSTox provides a high quality public chemistry resource for supporting improved predictive toxicology. | |
Disclaimer and Information on In-Vitro Research Products
Please be aware that all articles and product information presented on BenchChem are intended solely for informational purposes. The products available for purchase on BenchChem are specifically designed for in-vitro studies, which are conducted outside of living organisms. In-vitro studies, derived from the Latin term "in glass," involve experiments performed in controlled laboratory settings using cells or tissues. It is important to note that these products are not categorized as medicines or drugs, and they have not received approval from the FDA for the prevention, treatment, or cure of any medical condition, ailment, or disease. We must emphasize that any form of bodily introduction of these products into humans or animals is strictly prohibited by law. It is essential to adhere to these guidelines to ensure compliance with legal and ethical standards in research and experimentation.