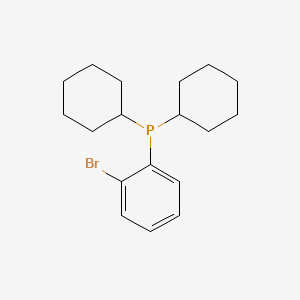
(2-Bromophenyl)dicyclohexylphosphine
- Click on QUICK INQUIRY to receive a quote from our team of experts.
- With the quality product at a COMPETITIVE price, you can focus more on your research.
Overview
Description
Preparation Methods
Synthetic Routes and Reaction Conditions: The synthesis of (2-Bromophenyl)dicyclohexylphosphine typically involves the reaction of (ortho-bromophenyl)dicyclohexylphosphine with n-butyllithium in diethyl ether and hexane at temperatures ranging from -30°C to 20°C under an inert atmosphere . This is followed by a reaction with 2-chloro-1,3-bis-(2,4,6-trimethylphenyl)[1,3,2]diazaphospholidine in diethyl ether and hexane at room temperature for 48 hours .
Industrial Production Methods: While specific industrial production methods are not detailed, the synthesis generally follows similar routes as laboratory preparation, scaled up to meet industrial demands. The use of inert atmospheres and controlled temperatures is crucial to ensure the purity and yield of the compound.
Chemical Reactions Analysis
Types of Reactions: (2-Bromophenyl)dicyclohexylphosphine is involved in various coupling reactions, including:
- Buchwald-Hartwig Cross Coupling Reaction
- Heck Reaction
- Hiyama Coupling
- Negishi Coupling
- Sonogashira Coupling
- Stille Coupling
- Suzuki-Miyaura Coupling
Common Reagents and Conditions: These reactions typically require palladium or nickel catalysts and are conducted under inert atmospheres. Solvents like toluene, THF, and diethyl ether are commonly used, and temperatures can range from room temperature to elevated temperatures depending on the specific reaction.
Major Products: The major products formed from these reactions are often complex organic molecules, including biaryl compounds, which are valuable in pharmaceuticals, agrochemicals, and materials science .
Scientific Research Applications
(2-Bromophenyl)dicyclohexylphosphine is widely used in scientific research due to its versatility as a ligand in various coupling reactions. Its applications include:
- Chemistry: Used in the synthesis of complex organic molecules and polymers.
- Biology: Employed in the modification of biomolecules for research purposes.
- Medicine: Utilized in the development of pharmaceutical intermediates and active compounds.
- Industry: Applied in the production of fine chemicals and materials .
Mechanism of Action
The mechanism of action of (2-Bromophenyl)dicyclohexylphosphine primarily involves its role as a ligand in catalytic cycles. It coordinates with metal centers (e.g., palladium or nickel) to facilitate the formation of carbon-carbon and carbon-heteroatom bonds. This coordination enhances the reactivity and selectivity of the metal catalysts, enabling efficient coupling reactions .
Comparison with Similar Compounds
- (2-Bromophenyl)diphenylphosphine
- 2-(Di-p-tolylphosphino)benzaldehyde
- Chlorodicyclohexylphosphine
Comparison: (2-Bromophenyl)dicyclohexylphosphine is unique due to its specific steric and electronic properties imparted by the dicyclohexyl groups. These properties make it particularly effective in certain coupling reactions compared to its analogs, which may have different substituents and thus different reactivity and selectivity profiles .
Properties
IUPAC Name |
(2-bromophenyl)-dicyclohexylphosphane |
Source
|
---|---|---|
Details | Computed by Lexichem TK 2.7.0 (PubChem release 2021.10.14) | |
Source | PubChem | |
URL | https://pubchem.ncbi.nlm.nih.gov | |
Description | Data deposited in or computed by PubChem | |
InChI |
InChI=1S/C18H26BrP/c19-17-13-7-8-14-18(17)20(15-9-3-1-4-10-15)16-11-5-2-6-12-16/h7-8,13-16H,1-6,9-12H2 |
Source
|
Details | Computed by InChI 1.0.6 (PubChem release 2021.10.14) | |
Source | PubChem | |
URL | https://pubchem.ncbi.nlm.nih.gov | |
Description | Data deposited in or computed by PubChem | |
InChI Key |
QFPIIICNJHDAKC-UHFFFAOYSA-N |
Source
|
Details | Computed by InChI 1.0.6 (PubChem release 2021.10.14) | |
Source | PubChem | |
URL | https://pubchem.ncbi.nlm.nih.gov | |
Description | Data deposited in or computed by PubChem | |
Canonical SMILES |
C1CCC(CC1)P(C2CCCCC2)C3=CC=CC=C3Br |
Source
|
Details | Computed by OEChem 2.3.0 (PubChem release 2021.10.14) | |
Source | PubChem | |
URL | https://pubchem.ncbi.nlm.nih.gov | |
Description | Data deposited in or computed by PubChem | |
Molecular Formula |
C18H26BrP |
Source
|
Details | Computed by PubChem 2.2 (PubChem release 2021.10.14) | |
Source | PubChem | |
URL | https://pubchem.ncbi.nlm.nih.gov | |
Description | Data deposited in or computed by PubChem | |
Molecular Weight |
353.3 g/mol |
Source
|
Details | Computed by PubChem 2.2 (PubChem release 2021.10.14) | |
Source | PubChem | |
URL | https://pubchem.ncbi.nlm.nih.gov | |
Description | Data deposited in or computed by PubChem | |
CAS No. |
757958-40-0 |
Source
|
Record name | 757958-40-0 | |
Source | European Chemicals Agency (ECHA) | |
URL | https://echa.europa.eu/information-on-chemicals | |
Description | The European Chemicals Agency (ECHA) is an agency of the European Union which is the driving force among regulatory authorities in implementing the EU's groundbreaking chemicals legislation for the benefit of human health and the environment as well as for innovation and competitiveness. | |
Explanation | Use of the information, documents and data from the ECHA website is subject to the terms and conditions of this Legal Notice, and subject to other binding limitations provided for under applicable law, the information, documents and data made available on the ECHA website may be reproduced, distributed and/or used, totally or in part, for non-commercial purposes provided that ECHA is acknowledged as the source: "Source: European Chemicals Agency, http://echa.europa.eu/". Such acknowledgement must be included in each copy of the material. ECHA permits and encourages organisations and individuals to create links to the ECHA website under the following cumulative conditions: Links can only be made to webpages that provide a link to the Legal Notice page. | |
Disclaimer and Information on In-Vitro Research Products
Please be aware that all articles and product information presented on BenchChem are intended solely for informational purposes. The products available for purchase on BenchChem are specifically designed for in-vitro studies, which are conducted outside of living organisms. In-vitro studies, derived from the Latin term "in glass," involve experiments performed in controlled laboratory settings using cells or tissues. It is important to note that these products are not categorized as medicines or drugs, and they have not received approval from the FDA for the prevention, treatment, or cure of any medical condition, ailment, or disease. We must emphasize that any form of bodily introduction of these products into humans or animals is strictly prohibited by law. It is essential to adhere to these guidelines to ensure compliance with legal and ethical standards in research and experimentation.