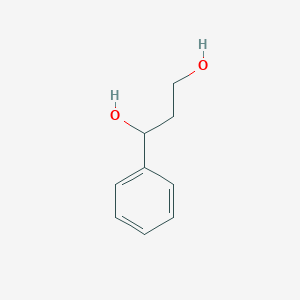
1-Phenylpropane-1,3-diol
Overview
Description
1-Phenylpropane-1,3-diol is an organic compound with the molecular formula C9H12O2. It is a type of diol, which means it contains two hydroxyl groups (-OH) attached to a propane chain with a phenyl group (C6H5) attached to the first carbon atom. This compound is known for its applications in various fields, including organic synthesis and pharmaceuticals .
Mechanism of Action
Target of Action
Similar compounds have been shown to interact with various enzymes such as pancreatic lipase .
Mode of Action
It’s known that phenylpropanoids, a class of compounds to which 1-phenylpropane-1,3-diol belongs, can undergo electrophilic aromatic substitution . This involves the formation of a sigma-bond to the benzene ring, generating a positively charged intermediate, followed by the removal of a proton, yielding a substituted benzene ring .
Biochemical Pathways
This compound is part of the phenylpropanoid class of compounds, which are synthesized by plants from phenylalanine or tyrosine through a series of enzymatic reactions . Phenylpropanoids contribute to all aspects of plant responses toward biotic and abiotic stimuli . They are key mediators of the plants’ resistance toward pests and are indicators of plant stress responses upon variation of light or mineral treatment .
Pharmacokinetics
Its molecular weight is 15219 g/mol , which may influence its absorption and distribution in the body.
Result of Action
It has been shown to be an inhibitor of pancreatic lipase and porcine pancreatic lipase with a potency similar to that of orlistat . This suggests that it may have potential applications in the treatment of conditions like obesity.
Biochemical Analysis
Biochemical Properties
1-Phenylpropane-1,3-diol plays a significant role in biochemical reactions, particularly in the context of its interactions with enzymes and proteins. It is known to interact with oxidoreductases, which are enzymes that catalyze oxidation-reduction reactions. These interactions often involve the hydroxyl groups of this compound, which can act as electron donors or acceptors in these reactions. Additionally, this compound can form hydrogen bonds with amino acid residues in proteins, influencing their structure and function .
Cellular Effects
This compound has been shown to affect various types of cells and cellular processes. It can influence cell signaling pathways by modulating the activity of kinases and phosphatases, which are enzymes that add or remove phosphate groups from proteins. This modulation can lead to changes in gene expression and cellular metabolism. For example, this compound has been observed to alter the expression of genes involved in oxidative stress responses, potentially enhancing the cell’s ability to cope with reactive oxygen species .
Molecular Mechanism
At the molecular level, this compound exerts its effects through several mechanisms. One key mechanism involves its binding interactions with specific biomolecules. The hydroxyl groups of this compound can form hydrogen bonds with the active sites of enzymes, leading to enzyme inhibition or activation. This compound can also influence gene expression by interacting with transcription factors, proteins that regulate the transcription of specific genes. These interactions can result in changes in the levels of mRNA and proteins, ultimately affecting cellular function .
Temporal Effects in Laboratory Settings
In laboratory settings, the effects of this compound can change over time. This compound is relatively stable under standard laboratory conditions, but it can degrade when exposed to high temperatures or strong acids. Over time, the degradation products of this compound can accumulate, potentially affecting its biological activity. Long-term studies have shown that prolonged exposure to this compound can lead to changes in cellular function, such as altered cell growth and differentiation .
Dosage Effects in Animal Models
The effects of this compound vary with different dosages in animal models. At low doses, this compound can enhance cellular metabolism and promote cell survival. At high doses, this compound can be toxic, leading to cell death and tissue damage. Studies in animal models have identified threshold doses above which adverse effects become significant. These studies also highlight the importance of careful dosage control to avoid toxic effects while maximizing the compound’s beneficial properties .
Metabolic Pathways
This compound is involved in several metabolic pathways, including those related to the metabolism of phenylpropanoids. This compound can be metabolized by enzymes such as cytochrome P450 oxidases, which introduce oxygen into the molecule, leading to its breakdown into smaller metabolites. These metabolic processes can affect the levels of various metabolites in the cell, influencing overall metabolic flux .
Transport and Distribution
Within cells and tissues, this compound is transported and distributed through interactions with specific transporters and binding proteins. These interactions can affect the localization and accumulation of the compound in different cellular compartments. For example, this compound can bind to albumin, a protein that transports various molecules in the bloodstream, facilitating its distribution to different tissues .
Subcellular Localization
The subcellular localization of this compound can influence its activity and function. This compound can be directed to specific cellular compartments through targeting signals or post-translational modifications. For instance, this compound may be localized to the mitochondria, where it can affect mitochondrial function and energy production. Understanding the subcellular localization of this compound is crucial for elucidating its role in cellular processes .
Preparation Methods
Synthetic Routes and Reaction Conditions: 1-Phenylpropane-1,3-diol can be synthesized through several methods. One common method involves the reduction of 1-phenyl-1,3-propanedione using sodium borohydride (NaBH4) or lithium aluminum hydride (LiAlH4) as reducing agents. The reaction typically occurs in an anhydrous solvent such as tetrahydrofuran (THF) or diethyl ether at low temperatures .
Industrial Production Methods: Industrial production of this compound often involves the catalytic hydrogenation of 1-phenyl-1,3-propanedione. This process uses a metal catalyst such as palladium on carbon (Pd/C) under hydrogen gas (H2) at elevated pressures and temperatures .
Chemical Reactions Analysis
Types of Reactions: 1-Phenylpropane-1,3-diol undergoes various chemical reactions, including:
Oxidation: The hydroxyl groups can be oxidized to form carbonyl compounds such as aldehydes or ketones using oxidizing agents like pyridinium chlorochromate (PCC) or Jones reagent.
Reduction: The compound can be further reduced to form alcohols using reducing agents like sodium borohydride (NaBH4) or lithium aluminum hydride (LiAlH4).
Common Reagents and Conditions:
Oxidation: Pyridinium chlorochromate (PCC), Jones reagent.
Reduction: Sodium borohydride (NaBH4), lithium aluminum hydride (LiAlH4).
Substitution: Thionyl chloride (SOCl2), phosphorus tribromide (PBr3).
Major Products:
Oxidation: Aldehydes, ketones.
Reduction: Alcohols.
Substitution: Halides, esters.
Scientific Research Applications
1-Phenylpropane-1,3-diol has several applications in scientific research:
Chemistry: It is used as an intermediate in the synthesis of various organic compounds, including pharmaceuticals and agrochemicals.
Biology: The compound is used in the study of enzyme-catalyzed reactions and metabolic pathways involving diols.
Medicine: It serves as a precursor for the synthesis of drugs and therapeutic agents.
Industry: It is used in the production of polymers, resins, and other industrial chemicals.
Comparison with Similar Compounds
2-Phenyl-1,3-propanediol: Similar structure but with the phenyl group attached to the second carbon atom.
1-Phenyl-1,2-propanediol: Similar structure but with the hydroxyl groups on the first and second carbon atoms.
1-Phenyl-2,3-propanediol: Similar structure but with the hydroxyl groups on the second and third carbon atoms.
Uniqueness: 1-Phenylpropane-1,3-diol is unique due to its specific arrangement of hydroxyl groups and the phenyl group, which imparts distinct chemical and physical properties. This unique structure allows it to participate in specific reactions and interactions that are not possible with its isomers .
Biological Activity
1-Phenylpropane-1,3-diol, also known as (S)-1-phenyl-1,3-propanediol, is a chiral compound with significant biological activity. This compound has garnered attention in various fields, including medicinal chemistry and pharmacology, due to its potential therapeutic applications. This article explores the biological activities associated with this compound, supported by research findings, case studies, and data tables.
This compound has the following chemical properties:
Property | Details |
---|---|
CAS Number | 96854-34-1 |
Molecular Formula | C₉H₁₂O₂ |
Molecular Weight | 152.19 g/mol |
Melting Point | 62.0°C to 66.0°C |
Purity | ≥ 98% |
Specific Rotation | -54.50° (22°C) |
The compound exists as a white crystalline solid and is soluble in various organic solvents, which facilitates its use in synthetic and biological applications.
Antioxidant Properties
Research has demonstrated that this compound exhibits significant antioxidant activity. A study conducted by showed that the compound effectively scavenges free radicals, thereby reducing oxidative stress in cellular models. The antioxidant capacity was measured using various assays, including DPPH and ABTS radical scavenging tests.
Anti-inflammatory Effects
In vitro studies have indicated that this compound possesses anti-inflammatory properties. It was found to inhibit the production of pro-inflammatory cytokines such as TNF-alpha and IL-6 in lipopolysaccharide (LPS)-stimulated macrophages. This suggests potential applications in treating inflammatory diseases .
Antimicrobial Activity
Several studies have explored the antimicrobial effects of this compound against various pathogens. For instance, it demonstrated inhibitory effects against Gram-positive bacteria such as Staphylococcus aureus and Gram-negative bacteria like Escherichia coli. The minimum inhibitory concentrations (MICs) were determined through standard broth dilution methods .
Case Study 1: Antioxidant Activity
A study published in the Journal of Medicinal Chemistry evaluated the antioxidant properties of various phenolic compounds, including this compound. The results indicated that this compound significantly reduced lipid peroxidation in rat liver homogenates compared to controls.
Case Study 2: Anti-inflammatory Mechanism
In a recent study examining the anti-inflammatory effects of several diols, researchers found that treatment with this compound led to a marked decrease in the expression of COX-2 and iNOS in RAW264.7 macrophages exposed to LPS .
Case Study 3: Antimicrobial Efficacy
A clinical trial investigated the efficacy of topical formulations containing this compound against skin infections caused by resistant bacterial strains. The results showed significant improvement in infection resolution rates compared to standard treatments.
Properties
IUPAC Name |
1-phenylpropane-1,3-diol | |
---|---|---|
Source | PubChem | |
URL | https://pubchem.ncbi.nlm.nih.gov | |
Description | Data deposited in or computed by PubChem | |
InChI |
InChI=1S/C9H12O2/c10-7-6-9(11)8-4-2-1-3-5-8/h1-5,9-11H,6-7H2 | |
Source | PubChem | |
URL | https://pubchem.ncbi.nlm.nih.gov | |
Description | Data deposited in or computed by PubChem | |
InChI Key |
RRVFYOSEKOTFOG-UHFFFAOYSA-N | |
Source | PubChem | |
URL | https://pubchem.ncbi.nlm.nih.gov | |
Description | Data deposited in or computed by PubChem | |
Canonical SMILES |
C1=CC=C(C=C1)C(CCO)O | |
Source | PubChem | |
URL | https://pubchem.ncbi.nlm.nih.gov | |
Description | Data deposited in or computed by PubChem | |
Molecular Formula |
C9H12O2 | |
Source | PubChem | |
URL | https://pubchem.ncbi.nlm.nih.gov | |
Description | Data deposited in or computed by PubChem | |
DSSTOX Substance ID |
DTXSID20341285 | |
Record name | 1-Phenyl-1,3-propanediol | |
Source | EPA DSSTox | |
URL | https://comptox.epa.gov/dashboard/DTXSID20341285 | |
Description | DSSTox provides a high quality public chemistry resource for supporting improved predictive toxicology. | |
Molecular Weight |
152.19 g/mol | |
Source | PubChem | |
URL | https://pubchem.ncbi.nlm.nih.gov | |
Description | Data deposited in or computed by PubChem | |
CAS No. |
4850-49-1 | |
Record name | 1-Phenyl-1,3-propanediol | |
Source | EPA DSSTox | |
URL | https://comptox.epa.gov/dashboard/DTXSID20341285 | |
Description | DSSTox provides a high quality public chemistry resource for supporting improved predictive toxicology. | |
Synthesis routes and methods
Procedure details
Retrosynthesis Analysis
AI-Powered Synthesis Planning: Our tool employs the Template_relevance Pistachio, Template_relevance Bkms_metabolic, Template_relevance Pistachio_ringbreaker, Template_relevance Reaxys, Template_relevance Reaxys_biocatalysis model, leveraging a vast database of chemical reactions to predict feasible synthetic routes.
One-Step Synthesis Focus: Specifically designed for one-step synthesis, it provides concise and direct routes for your target compounds, streamlining the synthesis process.
Accurate Predictions: Utilizing the extensive PISTACHIO, BKMS_METABOLIC, PISTACHIO_RINGBREAKER, REAXYS, REAXYS_BIOCATALYSIS database, our tool offers high-accuracy predictions, reflecting the latest in chemical research and data.
Strategy Settings
Precursor scoring | Relevance Heuristic |
---|---|
Min. plausibility | 0.01 |
Model | Template_relevance |
Template Set | Pistachio/Bkms_metabolic/Pistachio_ringbreaker/Reaxys/Reaxys_biocatalysis |
Top-N result to add to graph | 6 |
Feasible Synthetic Routes
Disclaimer and Information on In-Vitro Research Products
Please be aware that all articles and product information presented on BenchChem are intended solely for informational purposes. The products available for purchase on BenchChem are specifically designed for in-vitro studies, which are conducted outside of living organisms. In-vitro studies, derived from the Latin term "in glass," involve experiments performed in controlled laboratory settings using cells or tissues. It is important to note that these products are not categorized as medicines or drugs, and they have not received approval from the FDA for the prevention, treatment, or cure of any medical condition, ailment, or disease. We must emphasize that any form of bodily introduction of these products into humans or animals is strictly prohibited by law. It is essential to adhere to these guidelines to ensure compliance with legal and ethical standards in research and experimentation.