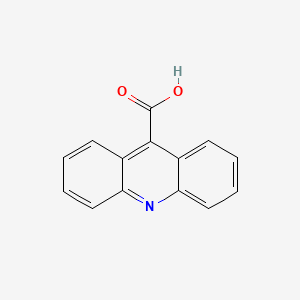
9-Acridinecarboxylic acid
Overview
Description
9-Acridinecarboxylic acid is a heterocyclic aromatic organic compound with the molecular formula C14H9NO2. It is known for its bright yellow to yellow-green crystalline appearance and is used in various scientific and industrial applications. This compound is particularly notable for its fluorescence properties, which make it useful in biochemical and analytical applications .
Mechanism of Action
Target of Action
The primary target of 9-Acridinecarboxylic acid is double-stranded DNA (dsDNA) . The compound acts as a DNA intercalator, inserting itself between the base pairs of the DNA helix . This interaction can disrupt the normal functioning of the DNA, affecting processes such as replication and transcription.
Mode of Action
This compound interacts with its target, dsDNA, by intercalation . Intercalation involves the insertion of the planar acridine ring system of the compound between the base pairs of the DNA helix . This can cause structural changes in the DNA, potentially leading to inhibition of DNA replication and transcription.
Result of Action
The interaction of this compound with dsDNA can lead to the inhibition of DNA replication and transcription . This can result in the inhibition of cell growth and proliferation.
Action Environment
The action of this compound can be influenced by environmental factors such as pH. For instance, the compound exhibits different fluorescence properties at acidic and alkaline conditions . At acidic conditions, it exists in a zwitterionic form with an emission centered at 480 nm, while at alkaline conditions, it exists in an anionic form with an emission centered at 430 nm . As the pH decreases, protonation of the heterocyclic N atom causes a red shift in the fluorescence peak and a decrease in fluorescence lifetime .
Biochemical Analysis
Biochemical Properties
9-Acridinecarboxylic acid plays a significant role in biochemical reactions, particularly as a DNA intercalator. It has been used to capture double-stranded DNAs (dsDNAs) duplex from solutions . The compound interacts with various enzymes and proteins, including α-amylase and α-glucosidase, and has been studied for its inhibition kinetics, structure-activity relationship (SAR), cytotoxicity, antitumor activity, and docking of ((benzyltriazolyl)methyl)acridine carboxamides . These interactions highlight the compound’s potential in biochemical research and therapeutic applications.
Cellular Effects
This compound influences various cellular processes, including cell signaling pathways, gene expression, and cellular metabolism. As a DNA intercalator, it can affect the transcription and replication processes by inserting itself between DNA base pairs, thereby disrupting the normal function of the DNA . This disruption can lead to changes in gene expression and cellular metabolism, potentially resulting in cytotoxic effects. The compound’s impact on cell signaling pathways and gene expression makes it a valuable tool for studying cellular processes and developing therapeutic agents.
Molecular Mechanism
The molecular mechanism of this compound involves its ability to intercalate into DNA, thereby affecting the DNA’s structure and function . This intercalation can inhibit or activate various enzymes involved in DNA replication and transcription. Additionally, the compound’s interactions with proteins and other biomolecules can lead to changes in gene expression and cellular function. The precise binding interactions and enzyme inhibition or activation mechanisms are crucial for understanding the compound’s effects at the molecular level.
Temporal Effects in Laboratory Settings
In laboratory settings, the effects of this compound can change over time. The compound’s stability and degradation are essential factors to consider when studying its long-term effects on cellular function. Studies have shown that this compound can maintain its stability under specific conditions, allowing for prolonged observation of its effects on cells . Degradation over time can lead to changes in its efficacy and potential cytotoxicity, highlighting the importance of monitoring its stability in experimental settings.
Dosage Effects in Animal Models
The effects of this compound vary with different dosages in animal models. At lower doses, the compound may exhibit minimal cytotoxic effects, while higher doses can lead to significant cytotoxicity and adverse effects . Studies have shown that there are threshold effects, where the compound’s impact on cellular function becomes more pronounced at specific dosage levels. Understanding these dosage effects is crucial for determining the compound’s therapeutic potential and safety in animal models.
Metabolic Pathways
This compound is involved in various metabolic pathways, interacting with enzymes and cofactors that influence its metabolism . The compound’s effects on metabolic flux and metabolite levels are essential for understanding its role in cellular metabolism. Studies have shown that this compound can affect the activity of enzymes involved in glycolysis and other metabolic pathways, leading to changes in cellular energy production and overall metabolism.
Transport and Distribution
The transport and distribution of this compound within cells and tissues are critical for its biological activity . The compound interacts with transporters and binding proteins that facilitate its movement across cellular membranes and its localization within specific tissues. Understanding these interactions is essential for determining the compound’s efficacy and potential therapeutic applications.
Subcellular Localization
The subcellular localization of this compound plays a significant role in its activity and function . The compound can be directed to specific compartments or organelles within the cell through targeting signals or post-translational modifications. This localization can influence the compound’s interactions with biomolecules and its overall biological activity. Studies have shown that this compound can localize to the nucleus, where it exerts its effects on DNA and gene expression.
Preparation Methods
Synthetic Routes and Reaction Conditions: 9-Acridinecarboxylic acid can be synthesized through several methods. One common method involves the Pfitzinger reaction, which uses 2,4,6-trihydroxytoluene (methylphloroglucinol) as a starting material. This reaction typically involves the use of acidic or basic conditions to facilitate the formation of the acridine ring .
Industrial Production Methods: In industrial settings, the production of this compound often involves the bromination and azo-coupling reactions of intermediate compounds. These processes are optimized for large-scale production to ensure high yield and purity .
Chemical Reactions Analysis
Types of Reactions: 9-Acridinecarboxylic acid undergoes various chemical reactions, including:
Oxidation: This reaction can convert this compound into other derivatives, such as 9-acridinecarboxaldehyde.
Reduction: Reduction reactions can yield products like 9-acridinemethanol.
Substitution: Substitution reactions, particularly involving halogens, can produce compounds like 9-chloroacridine
Common Reagents and Conditions:
Oxidation: Common oxidizing agents include potassium permanganate and chromium trioxide.
Reduction: Reducing agents such as sodium borohydride or lithium aluminum hydride are often used.
Substitution: Halogenation reactions typically use reagents like chlorine or bromine under controlled conditions
Major Products:
Oxidation: 9-Acridinecarboxaldehyde
Reduction: 9-Acridinemethanol
Substitution: 9-Chloroacridine
Scientific Research Applications
9-Acridinecarboxylic acid has a wide range of applications in scientific research:
Chemistry: It is used as a building block for synthesizing various heterocyclic compounds.
Biology: The compound is employed in the synthesis of DNA intercalators, which are used to study DNA structure and function.
Medicine: Research into its derivatives has shown potential antitumor activity, making it a candidate for drug development.
Industry: It is used in the production of dyes and as a fluorescent marker in various analytical techniques .
Comparison with Similar Compounds
Acridine: A parent compound of 9-acridinecarboxylic acid, used in the synthesis of various derivatives.
9-Aminoacridine: Known for its antiseptic properties and used in microbiology.
9-Chloroacridine:
Uniqueness: this compound is unique due to its specific fluorescence properties and its ability to intercalate into DNA. These characteristics make it particularly valuable in biochemical research and as a precursor for synthesizing other biologically active compounds .
Properties
IUPAC Name |
acridine-9-carboxylic acid | |
---|---|---|
Source | PubChem | |
URL | https://pubchem.ncbi.nlm.nih.gov | |
Description | Data deposited in or computed by PubChem | |
InChI |
InChI=1S/C14H9NO2/c16-14(17)13-9-5-1-3-7-11(9)15-12-8-4-2-6-10(12)13/h1-8H,(H,16,17) | |
Source | PubChem | |
URL | https://pubchem.ncbi.nlm.nih.gov | |
Description | Data deposited in or computed by PubChem | |
InChI Key |
IYRYQBAAHMBIFT-UHFFFAOYSA-N | |
Source | PubChem | |
URL | https://pubchem.ncbi.nlm.nih.gov | |
Description | Data deposited in or computed by PubChem | |
Canonical SMILES |
C1=CC=C2C(=C1)C(=C3C=CC=CC3=N2)C(=O)O | |
Source | PubChem | |
URL | https://pubchem.ncbi.nlm.nih.gov | |
Description | Data deposited in or computed by PubChem | |
Molecular Formula |
C14H9NO2 | |
Source | PubChem | |
URL | https://pubchem.ncbi.nlm.nih.gov | |
Description | Data deposited in or computed by PubChem | |
DSSTOX Substance ID |
DTXSID90201524 | |
Record name | 9-Acridinecarboxylic acid | |
Source | EPA DSSTox | |
URL | https://comptox.epa.gov/dashboard/DTXSID90201524 | |
Description | DSSTox provides a high quality public chemistry resource for supporting improved predictive toxicology. | |
Molecular Weight |
223.23 g/mol | |
Source | PubChem | |
URL | https://pubchem.ncbi.nlm.nih.gov | |
Description | Data deposited in or computed by PubChem | |
CAS No. |
5336-90-3 | |
Record name | 9-Acridinecarboxylic acid | |
Source | ChemIDplus | |
URL | https://pubchem.ncbi.nlm.nih.gov/substance/?source=chemidplus&sourceid=0005336903 | |
Description | ChemIDplus is a free, web search system that provides access to the structure and nomenclature authority files used for the identification of chemical substances cited in National Library of Medicine (NLM) databases, including the TOXNET system. | |
Record name | 9-Acridinecarboxylic acid | |
Source | DTP/NCI | |
URL | https://dtp.cancer.gov/dtpstandard/servlet/dwindex?searchtype=NSC&outputformat=html&searchlist=386 | |
Description | The NCI Development Therapeutics Program (DTP) provides services and resources to the academic and private-sector research communities worldwide to facilitate the discovery and development of new cancer therapeutic agents. | |
Explanation | Unless otherwise indicated, all text within NCI products is free of copyright and may be reused without our permission. Credit the National Cancer Institute as the source. | |
Record name | 9-Acridinecarboxylic acid | |
Source | EPA DSSTox | |
URL | https://comptox.epa.gov/dashboard/DTXSID90201524 | |
Description | DSSTox provides a high quality public chemistry resource for supporting improved predictive toxicology. | |
Record name | 5336-90-3 | |
Source | European Chemicals Agency (ECHA) | |
URL | https://echa.europa.eu/information-on-chemicals | |
Description | The European Chemicals Agency (ECHA) is an agency of the European Union which is the driving force among regulatory authorities in implementing the EU's groundbreaking chemicals legislation for the benefit of human health and the environment as well as for innovation and competitiveness. | |
Explanation | Use of the information, documents and data from the ECHA website is subject to the terms and conditions of this Legal Notice, and subject to other binding limitations provided for under applicable law, the information, documents and data made available on the ECHA website may be reproduced, distributed and/or used, totally or in part, for non-commercial purposes provided that ECHA is acknowledged as the source: "Source: European Chemicals Agency, http://echa.europa.eu/". Such acknowledgement must be included in each copy of the material. ECHA permits and encourages organisations and individuals to create links to the ECHA website under the following cumulative conditions: Links can only be made to webpages that provide a link to the Legal Notice page. | |
Synthesis routes and methods I
Procedure details
Synthesis routes and methods II
Procedure details
Retrosynthesis Analysis
AI-Powered Synthesis Planning: Our tool employs the Template_relevance Pistachio, Template_relevance Bkms_metabolic, Template_relevance Pistachio_ringbreaker, Template_relevance Reaxys, Template_relevance Reaxys_biocatalysis model, leveraging a vast database of chemical reactions to predict feasible synthetic routes.
One-Step Synthesis Focus: Specifically designed for one-step synthesis, it provides concise and direct routes for your target compounds, streamlining the synthesis process.
Accurate Predictions: Utilizing the extensive PISTACHIO, BKMS_METABOLIC, PISTACHIO_RINGBREAKER, REAXYS, REAXYS_BIOCATALYSIS database, our tool offers high-accuracy predictions, reflecting the latest in chemical research and data.
Strategy Settings
Precursor scoring | Relevance Heuristic |
---|---|
Min. plausibility | 0.01 |
Model | Template_relevance |
Template Set | Pistachio/Bkms_metabolic/Pistachio_ringbreaker/Reaxys/Reaxys_biocatalysis |
Top-N result to add to graph | 6 |
Feasible Synthetic Routes
Disclaimer and Information on In-Vitro Research Products
Please be aware that all articles and product information presented on BenchChem are intended solely for informational purposes. The products available for purchase on BenchChem are specifically designed for in-vitro studies, which are conducted outside of living organisms. In-vitro studies, derived from the Latin term "in glass," involve experiments performed in controlled laboratory settings using cells or tissues. It is important to note that these products are not categorized as medicines or drugs, and they have not received approval from the FDA for the prevention, treatment, or cure of any medical condition, ailment, or disease. We must emphasize that any form of bodily introduction of these products into humans or animals is strictly prohibited by law. It is essential to adhere to these guidelines to ensure compliance with legal and ethical standards in research and experimentation.