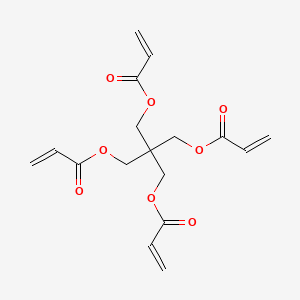
Pentaerythritol tetraacrylate
Overview
Description
Pentaerythritol tetraacrylate is an organic compound known for its tetrafunctional acrylate ester structure. It is widely used as a monomer in the manufacture of polymers. This compound is typically supplied with a polymerization inhibitor, such as monomethyl ether hydroquinone, to prevent premature polymerization .
Mechanism of Action
Target of Action
Pentaerythritol Tetraacrylate (PET4A) is primarily used as a cross-linker in the synthesis of polymers . It interacts with other monomers to form a polymer network, thereby enhancing the properties of the resulting material .
Mode of Action
PET4A’s mode of action is through its acrylate group functionality, which enables the molecule to undergo the Michael reaction with amines . This reaction is a type of conjugate addition, which is a critical step in the cross-linking process .
Biochemical Pathways
PET4A is involved in the synthesis of self-matting crosslinked acrylate coatings (PETs) . The cross-linking process, facilitated by PET4A, affects the gloss value of the PET films . The gloss value decreases as the amount of the cross-linker (PET4A) increases .
Result of Action
The primary result of PET4A’s action is the formation of crosslinked acrylate coatings with reduced gloss . These coatings exhibit excellent thermal stability and storage stability . They also provide a transparency of 84% with only 5 wt% of PET4A addition and exhibit a gloss of 5.0 (60°), illustrating the advantage of using PETs in low-gloss aqueous coatings .
Action Environment
The action of PET4A is influenced by several factors, including phase separation, cross-linking density, the film-forming temperature, and the film-forming thickness . These factors can affect the glossiness of the resulting PET films . .
Biochemical Analysis
Biochemical Properties
Pentaerythritol tetraacrylate plays a significant role in biochemical reactions due to its acrylate group functionality. This functionality enables the molecule to participate in the Michael reaction with amines, which is crucial in epoxy chemistry for reducing cure time . The compound interacts with various enzymes and proteins, including those involved in polymerization processes. The high cross-link density conferred by its four functional acrylate groups makes it a valuable crosslinking agent in biochemical applications .
Cellular Effects
This compound influences various cellular processes, including cell signaling pathways, gene expression, and cellular metabolism. It has been observed to form micelles and block copolymers, which can affect cell function by altering the cellular environment . The compound’s ability to participate in the Michael reaction with amines can also impact cellular signaling pathways and gene expression by modifying proteins and other biomolecules .
Molecular Mechanism
At the molecular level, this compound exerts its effects through binding interactions with biomolecules and enzyme inhibition or activation. The acrylate groups enable the molecule to form covalent bonds with amines, leading to the formation of stable complexes that can alter enzyme activity and gene expression . This mechanism is particularly important in epoxy chemistry, where the compound’s ability to reduce cure time is leveraged .
Temporal Effects in Laboratory Settings
In laboratory settings, the effects of this compound can change over time due to its stability and degradation properties. The compound is nearly always supplied with an added polymerization inhibitor, such as monomethyl ether hydroquinone, to prevent premature polymerization . Long-term studies have shown that the compound can maintain its functionality over extended periods, although its stability may be influenced by environmental factors such as temperature and pH .
Dosage Effects in Animal Models
The effects of this compound vary with different dosages in animal models. At low doses, the compound can effectively crosslink polymers without causing significant adverse effects. At high doses, it may exhibit toxic effects, including irritation and sensitization . Studies have shown that the compound’s impact on cellular function and metabolism can be dose-dependent, with higher doses potentially leading to more pronounced effects .
Metabolic Pathways
This compound is involved in various metabolic pathways, particularly those related to polymerization and crosslinking. The compound interacts with enzymes and cofactors involved in these processes, influencing metabolic flux and metabolite levels . Its ability to form stable complexes with amines through the Michael reaction is a key aspect of its metabolic activity .
Transport and Distribution
Within cells and tissues, this compound is transported and distributed through interactions with transporters and binding proteins. These interactions can affect the compound’s localization and accumulation, influencing its overall activity and function . The compound’s hydrophobic nature may also play a role in its distribution within cellular membranes and other hydrophobic environments .
Subcellular Localization
This compound’s subcellular localization is influenced by its targeting signals and post-translational modifications. The compound can be directed to specific compartments or organelles within the cell, where it exerts its effects on cellular processes . Its ability to form stable complexes with amines may also contribute to its localization and activity within the cell .
Preparation Methods
Synthetic Routes and Reaction Conditions: Pentaerythritol tetraacrylate is synthesized through the esterification of pentaerythritol with acrylic acid. The reaction typically involves the use of a catalyst, such as para-toluene sulfonic acid, and a solvent like toluene. The reaction system is maintained under reflux conditions to facilitate the removal of water, which drives the esterification to completion .
Industrial Production Methods: In industrial settings, the preparation of this compound involves adding pentaerythritol, acrylic acid, a solvent, a catalyst, and a polymerization inhibitor to a reactor. The reaction mixture is refluxed at a controlled temperature until the desired molar ratio of pentaerythritol triacrylate to this compound is achieved. The reaction mixture is then cooled, washed with water and alkali, and subjected to pressure-reducing distillation to obtain the final product .
Chemical Reactions Analysis
Types of Reactions: Pentaerythritol tetraacrylate undergoes various chemical reactions, including:
Polymerization: It can polymerize to form high cross-link density polymers.
Michael Addition: The acrylate groups can participate in Michael addition reactions with amines.
Common Reagents and Conditions:
Polymerization: Initiated by free radicals, UV light, or heat.
Michael Addition: Typically involves amines under mild conditions.
Major Products:
Polymeric Networks: Formed through polymerization.
Aminated Derivatives: Resulting from Michael addition reactions.
Scientific Research Applications
Pentaerythritol tetraacrylate has diverse applications in scientific research:
Polymeric Synthesis: Used to form micelles and block copolymers.
Epoxy Resin Chemistry: Reduces cure time in epoxy formulations.
UV-Curable Coatings: Enhances mechanical properties and performance.
Pharmaceuticals: Utilized in drug delivery systems and other medical applications.
Cementitious Systems: Improves the performance of polycarboxylate superplasticizers in concrete.
Comparison with Similar Compounds
- 1,6-Hexanediol Diacrylate
- Trimethylolpropane Triacrylate
- Acrylic Acid
Comparison: Pentaerythritol tetraacrylate stands out due to its tetrafunctional nature, which provides higher cross-link density compared to compounds like 1,6-hexanediol diacrylate and trimethylolpropane triacrylate. This unique feature makes it particularly valuable in applications requiring robust polymeric networks .
Properties
IUPAC Name |
[3-prop-2-enoyloxy-2,2-bis(prop-2-enoyloxymethyl)propyl] prop-2-enoate | |
---|---|---|
Source | PubChem | |
URL | https://pubchem.ncbi.nlm.nih.gov | |
Description | Data deposited in or computed by PubChem | |
InChI |
InChI=1S/C17H20O8/c1-5-13(18)22-9-17(10-23-14(19)6-2,11-24-15(20)7-3)12-25-16(21)8-4/h5-8H,1-4,9-12H2 | |
Source | PubChem | |
URL | https://pubchem.ncbi.nlm.nih.gov | |
Description | Data deposited in or computed by PubChem | |
InChI Key |
KNSXNCFKSZZHEA-UHFFFAOYSA-N | |
Source | PubChem | |
URL | https://pubchem.ncbi.nlm.nih.gov | |
Description | Data deposited in or computed by PubChem | |
Canonical SMILES |
C=CC(=O)OCC(COC(=O)C=C)(COC(=O)C=C)COC(=O)C=C | |
Source | PubChem | |
URL | https://pubchem.ncbi.nlm.nih.gov | |
Description | Data deposited in or computed by PubChem | |
Molecular Formula |
C17H20O8 | |
Source | PubChem | |
URL | https://pubchem.ncbi.nlm.nih.gov | |
Description | Data deposited in or computed by PubChem | |
Related CAS |
57592-66-2 | |
Record name | 2-Propenoic acid, 1,1′-[2,2-bis[[(1-oxo-2-propen-1-yl)oxy]methyl]-1,3-propanediyl] ester, homopolymer | |
Source | CAS Common Chemistry | |
URL | https://commonchemistry.cas.org/detail?cas_rn=57592-66-2 | |
Description | CAS Common Chemistry is an open community resource for accessing chemical information. Nearly 500,000 chemical substances from CAS REGISTRY cover areas of community interest, including common and frequently regulated chemicals, and those relevant to high school and undergraduate chemistry classes. This chemical information, curated by our expert scientists, is provided in alignment with our mission as a division of the American Chemical Society. | |
Explanation | The data from CAS Common Chemistry is provided under a CC-BY-NC 4.0 license, unless otherwise stated. | |
DSSTOX Substance ID |
DTXSID8042264 | |
Record name | Pentaerythritol tetraacrylate | |
Source | EPA DSSTox | |
URL | https://comptox.epa.gov/dashboard/DTXSID8042264 | |
Description | DSSTox provides a high quality public chemistry resource for supporting improved predictive toxicology. | |
Molecular Weight |
352.3 g/mol | |
Source | PubChem | |
URL | https://pubchem.ncbi.nlm.nih.gov | |
Description | Data deposited in or computed by PubChem | |
Physical Description |
Liquid; NKRA, Colorless viscous liquid; [Sigma-Aldrich MSDS] | |
Record name | 2-Propenoic acid, 1,1'-[2,2-bis[[(1-oxo-2-propen-1-yl)oxy]methyl]-1,3-propanediyl] ester | |
Source | EPA Chemicals under the TSCA | |
URL | https://www.epa.gov/chemicals-under-tsca | |
Description | EPA Chemicals under the Toxic Substances Control Act (TSCA) collection contains information on chemicals and their regulations under TSCA, including non-confidential content from the TSCA Chemical Substance Inventory and Chemical Data Reporting. | |
Record name | Pentaerythritol tetraacrylate | |
Source | Haz-Map, Information on Hazardous Chemicals and Occupational Diseases | |
URL | https://haz-map.com/Agents/21432 | |
Description | Haz-Map® is an occupational health database designed for health and safety professionals and for consumers seeking information about the adverse effects of workplace exposures to chemical and biological agents. | |
Explanation | Copyright (c) 2022 Haz-Map(R). All rights reserved. Unless otherwise indicated, all materials from Haz-Map are copyrighted by Haz-Map(R). No part of these materials, either text or image may be used for any purpose other than for personal use. Therefore, reproduction, modification, storage in a retrieval system or retransmission, in any form or by any means, electronic, mechanical or otherwise, for reasons other than personal use, is strictly prohibited without prior written permission. | |
CAS No. |
4986-89-4 | |
Record name | Pentaerythritol tetraacrylate | |
Source | CAS Common Chemistry | |
URL | https://commonchemistry.cas.org/detail?cas_rn=4986-89-4 | |
Description | CAS Common Chemistry is an open community resource for accessing chemical information. Nearly 500,000 chemical substances from CAS REGISTRY cover areas of community interest, including common and frequently regulated chemicals, and those relevant to high school and undergraduate chemistry classes. This chemical information, curated by our expert scientists, is provided in alignment with our mission as a division of the American Chemical Society. | |
Explanation | The data from CAS Common Chemistry is provided under a CC-BY-NC 4.0 license, unless otherwise stated. | |
Record name | Pentaerythritol tetraacrylate | |
Source | ChemIDplus | |
URL | https://pubchem.ncbi.nlm.nih.gov/substance/?source=chemidplus&sourceid=0004986894 | |
Description | ChemIDplus is a free, web search system that provides access to the structure and nomenclature authority files used for the identification of chemical substances cited in National Library of Medicine (NLM) databases, including the TOXNET system. | |
Record name | 2-Propenoic acid, 1,1'-[2,2-bis[[(1-oxo-2-propen-1-yl)oxy]methyl]-1,3-propanediyl] ester | |
Source | EPA Chemicals under the TSCA | |
URL | https://www.epa.gov/chemicals-under-tsca | |
Description | EPA Chemicals under the Toxic Substances Control Act (TSCA) collection contains information on chemicals and their regulations under TSCA, including non-confidential content from the TSCA Chemical Substance Inventory and Chemical Data Reporting. | |
Record name | Pentaerythritol tetraacrylate | |
Source | EPA DSSTox | |
URL | https://comptox.epa.gov/dashboard/DTXSID8042264 | |
Description | DSSTox provides a high quality public chemistry resource for supporting improved predictive toxicology. | |
Record name | 2,2-bis[[(1-oxoallyl)oxy]methyl]-1,3-propanediyl diacrylate | |
Source | European Chemicals Agency (ECHA) | |
URL | https://echa.europa.eu/substance-information/-/substanceinfo/100.023.313 | |
Description | The European Chemicals Agency (ECHA) is an agency of the European Union which is the driving force among regulatory authorities in implementing the EU's groundbreaking chemicals legislation for the benefit of human health and the environment as well as for innovation and competitiveness. | |
Explanation | Use of the information, documents and data from the ECHA website is subject to the terms and conditions of this Legal Notice, and subject to other binding limitations provided for under applicable law, the information, documents and data made available on the ECHA website may be reproduced, distributed and/or used, totally or in part, for non-commercial purposes provided that ECHA is acknowledged as the source: "Source: European Chemicals Agency, http://echa.europa.eu/". Such acknowledgement must be included in each copy of the material. ECHA permits and encourages organisations and individuals to create links to the ECHA website under the following cumulative conditions: Links can only be made to webpages that provide a link to the Legal Notice page. | |
Record name | PENTAERYTHRITYL TETRAACRYLATE | |
Source | FDA Global Substance Registration System (GSRS) | |
URL | https://gsrs.ncats.nih.gov/ginas/app/beta/substances/50LIA3DGN1 | |
Description | The FDA Global Substance Registration System (GSRS) enables the efficient and accurate exchange of information on what substances are in regulated products. Instead of relying on names, which vary across regulatory domains, countries, and regions, the GSRS knowledge base makes it possible for substances to be defined by standardized, scientific descriptions. | |
Explanation | Unless otherwise noted, the contents of the FDA website (www.fda.gov), both text and graphics, are not copyrighted. They are in the public domain and may be republished, reprinted and otherwise used freely by anyone without the need to obtain permission from FDA. Credit to the U.S. Food and Drug Administration as the source is appreciated but not required. | |
Retrosynthesis Analysis
AI-Powered Synthesis Planning: Our tool employs the Template_relevance Pistachio, Template_relevance Bkms_metabolic, Template_relevance Pistachio_ringbreaker, Template_relevance Reaxys, Template_relevance Reaxys_biocatalysis model, leveraging a vast database of chemical reactions to predict feasible synthetic routes.
One-Step Synthesis Focus: Specifically designed for one-step synthesis, it provides concise and direct routes for your target compounds, streamlining the synthesis process.
Accurate Predictions: Utilizing the extensive PISTACHIO, BKMS_METABOLIC, PISTACHIO_RINGBREAKER, REAXYS, REAXYS_BIOCATALYSIS database, our tool offers high-accuracy predictions, reflecting the latest in chemical research and data.
Strategy Settings
Precursor scoring | Relevance Heuristic |
---|---|
Min. plausibility | 0.01 |
Model | Template_relevance |
Template Set | Pistachio/Bkms_metabolic/Pistachio_ringbreaker/Reaxys/Reaxys_biocatalysis |
Top-N result to add to graph | 6 |
Feasible Synthetic Routes
Q1: What is the molecular formula and weight of Pentaerythritol tetraacrylate?
A1: this compound has a molecular formula of C17H20O8 and a molecular weight of 352.33 g/mol.
Q2: What spectroscopic techniques are used to characterize this compound?
A: Researchers commonly employ Fourier Transform Infrared Spectroscopy (FTIR) [, , , , ], Raman spectroscopy [, ], and Nuclear Magnetic Resonance (NMR) spectroscopy [, , ] to characterize the structure and monitor reactions involving PETA.
Q3: How does this compound influence the properties of polymer networks?
A: PETA's tetrafunctional nature allows it to act as a highly effective cross-linking agent. This cross-linking significantly impacts the mechanical properties of the resulting polymers, enhancing their hardness [], tensile strength [], and thermal stability [, ].
Q4: Can this compound be used to modify existing polymers?
A: Yes, PETA can be used to modify pre-existing polymers. For instance, researchers have successfully employed PETA as a cross-linking promoter in natural rubber/acrylonitrile-butadiene rubber latex blends []. This cross-linking enhanced the gel content and swelling resistance of the blend, improving its overall properties.
Q5: What is the role of this compound in creating self-matting coatings?
A: Studies have demonstrated the effectiveness of PETA in formulating self-matting crosslinked acrylate coatings []. As the PETA concentration increases, the gloss value of the resulting films decreases. This phenomenon is attributed to factors like phase separation, cross-linking density, and film-forming parameters.
Q6: How is this compound used in drug delivery systems?
A: Research highlights the potential of PETA in developing drug delivery systems (DDS). Studies show that UV-cured PEGDA scaffolds, modified with varying concentrations of PETA, demonstrate controlled release profiles for the antiseptic drug chlorhexidine [].
Q7: Can this compound be used to create stable nanoparticles for drug delivery?
A: Yes, PETA plays a crucial role in synthesizing thiolated nanoparticles with mucoadhesive properties []. These nanoparticles demonstrate potential for drug delivery applications due to their ability to adhere to mucosal surfaces.
Q8: How does this compound contribute to the development of solid-state lithium-sulfur batteries?
A: PETA serves as a key component in the development of advanced electrolytes for lithium-sulfur batteries. For example, researchers have incorporated PETA into solid polymer electrolytes (SPEs) for use in these batteries [, ]. The crosslinked PETA-based SPEs contribute to enhanced battery performance, including high initial discharge capacity and good rate capability, by acting as both a binder and an electrolyte within the cathode.
Q9: Can this compound facilitate the fabrication of 3D-printed porous carbon materials?
A: Yes, PETA plays a crucial role in fabricating 3D-printed porous carbon materials []. Its photopolymerizable nature, combined with divinylbenzene, enables the creation of mechanically stable structures with controlled porosity. This technique holds promise for various applications, including energy storage and catalysis.
Q10: What role does this compound play in the fabrication of Polymer Dispersed Liquid Crystal (PDLC) films?
A: Research indicates that PDLC films prepared with PETA exhibit interesting optical properties, including a permanent memory effect []. These films, with their ability to switch between opaque and transparent states, hold potential for applications in optical memory devices.
Q11: How do the reaction kinetics of this compound polymerization compare to other multifunctional monomers?
A: Studies comparing the polymerization kinetics of PETA to other multifunctional monomers like trimethylolpropane triacrylate reveal crucial differences in volume shrinkage and functional group conversion rates []. These differences highlight the impact of the number and type of functional groups on polymerization behavior.
Q12: What is the role of stimulated emission depletion in controlling this compound polymerization?
A: Research demonstrates that stimulated emission depletion can be effectively utilized to achieve ultrafast polymerization inhibition in PETA-based photoresists []. This finding paves the way for high-resolution three-dimensional nanolithography.
Q13: How is computational chemistry used to study this compound?
A: While the provided abstracts do not mention specific computational studies on PETA itself, they highlight the application of computational techniques like first-principle calculations [] and stochastic modeling [] to understand the behavior of PETA-containing systems. These approaches help elucidate reaction mechanisms, predict material properties, and guide experimental design.
Q14: What are the environmental considerations related to this compound?
A14: While the research abstracts don't specifically address the environmental impact of PETA, it's crucial to consider the potential environmental implications of its production, use, and disposal. Further research might be necessary to explore its biodegradability, potential for bioaccumulation, and strategies for sustainable manufacturing and waste management.
Q15: Are there alternative materials to this compound for specific applications?
A: The choice of alternative materials to PETA is highly dependent on the specific application. For instance, other multifunctional acrylates like trimethylolpropane triacrylate [], dipentaerythritol pentaacrylate [], or tris[2-(acryloyloxy)ethyl] isocyanurate [] might be considered depending on the desired properties of the final product.
Disclaimer and Information on In-Vitro Research Products
Please be aware that all articles and product information presented on BenchChem are intended solely for informational purposes. The products available for purchase on BenchChem are specifically designed for in-vitro studies, which are conducted outside of living organisms. In-vitro studies, derived from the Latin term "in glass," involve experiments performed in controlled laboratory settings using cells or tissues. It is important to note that these products are not categorized as medicines or drugs, and they have not received approval from the FDA for the prevention, treatment, or cure of any medical condition, ailment, or disease. We must emphasize that any form of bodily introduction of these products into humans or animals is strictly prohibited by law. It is essential to adhere to these guidelines to ensure compliance with legal and ethical standards in research and experimentation.