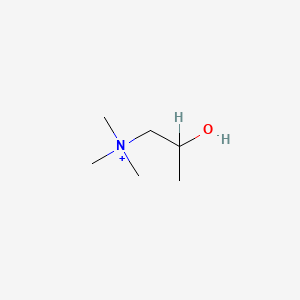
2-Methylcholine
Overview
Description
Preparation Methods
2-Methylcholine can be synthesized through various methods. One common synthetic route involves the reaction of choline with acetic anhydride to form acetylcholine, followed by methylation using methyl iodide to produce this compound . Industrial production methods typically involve the use of ion chromatography to determine and purify methacholine chloride, which is a related compound .
Chemical Reactions Analysis
2-Methylcholine undergoes several types of chemical reactions, including:
Oxidation: It can be oxidized to form corresponding oxides.
Reduction: Reduction reactions can convert it back to its precursor forms.
Substitution: It can undergo substitution reactions where the methyl group is replaced by other functional groups.
Common reagents used in these reactions include oxidizing agents like potassium permanganate and reducing agents like lithium aluminum hydride. The major products formed from these reactions depend on the specific conditions and reagents used .
Scientific Research Applications
2-Methylcholine has a wide range of scientific research applications:
Chemistry: It is used as a reagent in various chemical synthesis processes.
Biology: It is used to study the function of muscarinic receptors in biological systems.
Medicine: It is primarily used to diagnose bronchial hyperreactivity through the methacholine challenge test.
Industry: It is used in the production of various pharmaceuticals and diagnostic agents.
Mechanism of Action
2-Methylcholine exerts its effects by acting as a non-selective muscarinic receptor agonist. It binds to muscarinic receptors in the parasympathetic nervous system, leading to the activation of downstream calcium-dependent signaling pathways. This results in smooth muscle contraction and bronchoconstriction . The quaternary ammonium group in its structure is essential for its activity .
Comparison with Similar Compounds
2-Methylcholine is similar to other choline esters such as acetylcholine and methacholine. its unique structure with a methyl group on the beta carbon provides selectivity towards muscarinic receptors compared to nicotinic receptors . Other similar compounds include:
Acetylcholine: A neurotransmitter that acts on both muscarinic and nicotinic receptors.
Methacholine: A synthetic choline ester used in bronchial challenge tests.
This compound’s selectivity towards muscarinic receptors makes it particularly useful in diagnostic applications where specific activation of these receptors is required .
Properties
IUPAC Name |
2-hydroxypropyl(trimethyl)azanium | |
---|---|---|
Source | PubChem | |
URL | https://pubchem.ncbi.nlm.nih.gov | |
Description | Data deposited in or computed by PubChem | |
InChI |
InChI=1S/C6H16NO/c1-6(8)5-7(2,3)4/h6,8H,5H2,1-4H3/q+1 | |
Source | PubChem | |
URL | https://pubchem.ncbi.nlm.nih.gov | |
Description | Data deposited in or computed by PubChem | |
InChI Key |
JPKKMFOXWKNEEN-UHFFFAOYSA-N | |
Source | PubChem | |
URL | https://pubchem.ncbi.nlm.nih.gov | |
Description | Data deposited in or computed by PubChem | |
Canonical SMILES |
CC(C[N+](C)(C)C)O | |
Source | PubChem | |
URL | https://pubchem.ncbi.nlm.nih.gov | |
Description | Data deposited in or computed by PubChem | |
Molecular Formula |
C6H16NO+ | |
Source | PubChem | |
URL | https://pubchem.ncbi.nlm.nih.gov | |
Description | Data deposited in or computed by PubChem | |
Related CAS |
2382-43-6 (chloride), 62314-25-4 (formate salt), 70624-15-6 (cyanoacetic acid (1:1)salt) | |
Record name | beta-Methylcholine | |
Source | ChemIDplus | |
URL | https://pubchem.ncbi.nlm.nih.gov/substance/?source=chemidplus&sourceid=0007562870 | |
Description | ChemIDplus is a free, web search system that provides access to the structure and nomenclature authority files used for the identification of chemical substances cited in National Library of Medicine (NLM) databases, including the TOXNET system. | |
DSSTOX Substance ID |
DTXSID90864092 | |
Record name | 2-Hydroxy-N,N,N-trimethylpropan-1-aminium | |
Source | EPA DSSTox | |
URL | https://comptox.epa.gov/dashboard/DTXSID90864092 | |
Description | DSSTox provides a high quality public chemistry resource for supporting improved predictive toxicology. | |
Molecular Weight |
118.20 g/mol | |
Source | PubChem | |
URL | https://pubchem.ncbi.nlm.nih.gov | |
Description | Data deposited in or computed by PubChem | |
CAS No. |
7562-87-0 | |
Record name | β-Methylcholine | |
Source | CAS Common Chemistry | |
URL | https://commonchemistry.cas.org/detail?cas_rn=7562-87-0 | |
Description | CAS Common Chemistry is an open community resource for accessing chemical information. Nearly 500,000 chemical substances from CAS REGISTRY cover areas of community interest, including common and frequently regulated chemicals, and those relevant to high school and undergraduate chemistry classes. This chemical information, curated by our expert scientists, is provided in alignment with our mission as a division of the American Chemical Society. | |
Explanation | The data from CAS Common Chemistry is provided under a CC-BY-NC 4.0 license, unless otherwise stated. | |
Record name | beta-Methylcholine | |
Source | ChemIDplus | |
URL | https://pubchem.ncbi.nlm.nih.gov/substance/?source=chemidplus&sourceid=0007562870 | |
Description | ChemIDplus is a free, web search system that provides access to the structure and nomenclature authority files used for the identification of chemical substances cited in National Library of Medicine (NLM) databases, including the TOXNET system. | |
Record name | (2-hydroxypropyl)trimethylammonium | |
Source | European Chemicals Agency (ECHA) | |
URL | https://echa.europa.eu/substance-information/-/substanceinfo/100.028.598 | |
Description | The European Chemicals Agency (ECHA) is an agency of the European Union which is the driving force among regulatory authorities in implementing the EU's groundbreaking chemicals legislation for the benefit of human health and the environment as well as for innovation and competitiveness. | |
Explanation | Use of the information, documents and data from the ECHA website is subject to the terms and conditions of this Legal Notice, and subject to other binding limitations provided for under applicable law, the information, documents and data made available on the ECHA website may be reproduced, distributed and/or used, totally or in part, for non-commercial purposes provided that ECHA is acknowledged as the source: "Source: European Chemicals Agency, http://echa.europa.eu/". Such acknowledgement must be included in each copy of the material. ECHA permits and encourages organisations and individuals to create links to the ECHA website under the following cumulative conditions: Links can only be made to webpages that provide a link to the Legal Notice page. | |
Record name | .BETA.-METHYLCHOLINE | |
Source | FDA Global Substance Registration System (GSRS) | |
URL | https://gsrs.ncats.nih.gov/ginas/app/beta/substances/842Q90137O | |
Description | The FDA Global Substance Registration System (GSRS) enables the efficient and accurate exchange of information on what substances are in regulated products. Instead of relying on names, which vary across regulatory domains, countries, and regions, the GSRS knowledge base makes it possible for substances to be defined by standardized, scientific descriptions. | |
Explanation | Unless otherwise noted, the contents of the FDA website (www.fda.gov), both text and graphics, are not copyrighted. They are in the public domain and may be republished, reprinted and otherwise used freely by anyone without the need to obtain permission from FDA. Credit to the U.S. Food and Drug Administration as the source is appreciated but not required. | |
Retrosynthesis Analysis
AI-Powered Synthesis Planning: Our tool employs the Template_relevance Pistachio, Template_relevance Bkms_metabolic, Template_relevance Pistachio_ringbreaker, Template_relevance Reaxys, Template_relevance Reaxys_biocatalysis model, leveraging a vast database of chemical reactions to predict feasible synthetic routes.
One-Step Synthesis Focus: Specifically designed for one-step synthesis, it provides concise and direct routes for your target compounds, streamlining the synthesis process.
Accurate Predictions: Utilizing the extensive PISTACHIO, BKMS_METABOLIC, PISTACHIO_RINGBREAKER, REAXYS, REAXYS_BIOCATALYSIS database, our tool offers high-accuracy predictions, reflecting the latest in chemical research and data.
Strategy Settings
Precursor scoring | Relevance Heuristic |
---|---|
Min. plausibility | 0.01 |
Model | Template_relevance |
Template Set | Pistachio/Bkms_metabolic/Pistachio_ringbreaker/Reaxys/Reaxys_biocatalysis |
Top-N result to add to graph | 6 |
Feasible Synthetic Routes
Disclaimer and Information on In-Vitro Research Products
Please be aware that all articles and product information presented on BenchChem are intended solely for informational purposes. The products available for purchase on BenchChem are specifically designed for in-vitro studies, which are conducted outside of living organisms. In-vitro studies, derived from the Latin term "in glass," involve experiments performed in controlled laboratory settings using cells or tissues. It is important to note that these products are not categorized as medicines or drugs, and they have not received approval from the FDA for the prevention, treatment, or cure of any medical condition, ailment, or disease. We must emphasize that any form of bodily introduction of these products into humans or animals is strictly prohibited by law. It is essential to adhere to these guidelines to ensure compliance with legal and ethical standards in research and experimentation.