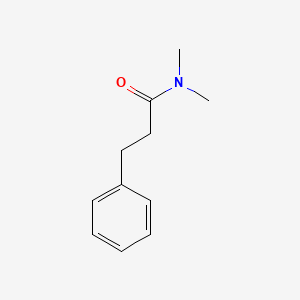
N,N-Dimethyl-3-phenylpropanamide
Overview
Description
N,N-Dimethyl-3-phenylpropanamide (CAS: Not explicitly provided; molecular formula: C₁₁H₁₅NO) is a tertiary aliphatic amide characterized by a phenyl group at the β-position and dimethylamino substituents on the nitrogen atom. It serves as a versatile intermediate in organic synthesis and pharmaceutical research.
Preparation Methods
Classical Acid Chloride Route
Reaction Mechanism and Procedure
The traditional method involves converting 3-phenylpropanoic acid to its corresponding acid chloride, followed by reaction with dimethylamine. The process begins with treating 3-phenylpropanoic acid with thionyl chloride (SOCl₂) at 60–80°C for 2–4 hours to form 3-phenylpropanoyl chloride. Subsequent addition of dimethylamine in anhydrous tetrahydrofuran (THF) at 0–5°C yields N,N-dimethyl-3-phenylpropanamide .
Optimization and Challenges
Key parameters include stoichiometric control of SOCl₂ (1.2–1.5 equivalents) and dimethylamine (2.0 equivalents). Excess SOCl₂ ensures complete acid conversion but necessitates careful quenching to prevent side reactions. The method achieves yields of 70–85%, though it faces criticism for using hazardous reagents (SOCl₂) and generating HCl gas .
CDI-Mediated Direct Amidation
Green Chemistry Approach
A modern alternative employs 1,1′-carbonyldiimidazole (CDI) as a coupling agent, enabling direct amidation of 3-phenylpropanoic acid with dimethylamine. In this one-pot synthesis, CDI (1.1 equivalents) activates the carboxylic acid in N,N-dimethylacetamide (DMAc) at 160–165°C for 2–24 hours. The activated intermediate reacts with dimethylamine to form the target amide .
Advantages Over Classical Methods
This method eliminates acid chloride handling and reduces solvent waste. Yields improve to 85–92%, with higher purity due to fewer byproducts. DMAc serves dual roles as solvent and dimethylamine source, enhancing atom economy .
Catalytic Hydrogenation of Nitriles
Two-Step Synthesis
A patent-derived approach involves Friedel-Crafts alkylation of cinnamonitrile with benzene using AlCl₃ as a catalyst, producing 3,3-diphenylpropanenitrile. Catalytic hydrogenation (H₂, 50–100 psi, Pd/C) reduces the nitrile to 3,3-diphenylpropylamine, which undergoes methylation and hydrolysis to yield this compound .
Industrial Scalability
This method is favored for large-scale production due to robust catalysts and reusable solvents (e.g., toluene). However, the multi-step process requires precise control of hydrogenation conditions to avoid over-reduction .
Comparative Analysis of Synthetic Routes
Method | Reagents | Yield | Purity | Environmental Impact |
---|---|---|---|---|
Acid Chloride Route | SOCl₂, (CH₃)₂NH | 70–85% | 90–95% | High (HCl gas, SOCl₂) |
CDI-Mediated Amidation | CDI, DMAc | 85–92% | 95–98% | Low (solvent recycling) |
Catalytic Hydrogenation | AlCl₃, H₂, Pd/C | 65–75% | 85–90% | Moderate (metal waste) |
Emerging Techniques and Innovations
Microwave-Assisted Synthesis
Recent studies explore microwave irradiation to accelerate CDI-mediated amidation, reducing reaction times from 24 hours to 30–60 minutes. Initial trials report yields comparable to conventional heating (88–90%) .
Enzymatic Catalysis
Pilot-scale experiments using lipases (e.g., Candida antarctica) demonstrate selective amidation under mild conditions (40°C, pH 7.5). While yields remain lower (50–60%), this method avoids toxic reagents and aligns with green chemistry principles .
Industrial Production and Purification
Large-Scale Reactor Design
Industrial facilities utilize continuous-flow reactors for CDI-mediated reactions, enhancing heat transfer and reducing batch variability. Post-reaction purification involves distillation under reduced pressure (0.1–0.5 mmHg) to isolate the amide (>99% purity) .
Waste Management Strategies
SOCl₂-based methods require HCl scrubbers and neutralization tanks, whereas CDI routes generate imidazole byproducts, which are reclaimed via crystallization. Patent CN102381993A highlights solvent recovery systems that reduce DMAc consumption by 40% .
Chemical Reactions Analysis
Types of Reactions
N,N-Dimethyl-3-phenylpropanamide undergoes various chemical reactions, including:
Oxidation: The compound can be oxidized to form corresponding carboxylic acids.
Reduction: Reduction reactions can convert the amide group to an amine.
Substitution: The amide group can participate in nucleophilic substitution reactions.
Common Reagents and Conditions
Oxidation: Common oxidizing agents include potassium permanganate (KMnO4) and chromium trioxide (CrO3).
Reduction: Reducing agents like lithium aluminum hydride (LiAlH4) are often used.
Substitution: Nucleophiles such as hydroxide ions (OH-) can be used in substitution reactions.
Major Products Formed
Oxidation: Formation of carboxylic acids.
Reduction: Formation of amines.
Substitution: Formation of substituted amides or other derivatives.
Scientific Research Applications
Organic Synthesis
N,N-Dimethyl-3-phenylpropanamide serves as an important intermediate in the synthesis of various organic compounds, particularly pharmaceuticals and agrochemicals. It is utilized in reactions such as radical condensation with benzylic alcohols to produce 3-arylpropanamides, showcasing its utility in developing new synthetic methodologies .
Research indicates that this compound exhibits potential biological activities. It has been studied for:
- Enzyme Inhibition : The compound may interact with specific enzymes, modulating their activity, which is crucial for drug development and understanding metabolic pathways.
- Therapeutic Effects : Investigations into its anti-inflammatory and analgesic properties suggest potential applications in treating inflammatory diseases .
Anti-inflammatory Activity
A study assessed the anti-inflammatory effects of this compound using a murine model of arthritis. The results indicated a significant reduction in pro-inflammatory cytokines, supporting its potential use as an anti-inflammatory agent.
Anticancer Research
In another study focusing on ovarian cancer models, this compound demonstrated the ability to inhibit tumor growth by inducing apoptosis and inhibiting angiogenesis. This finding highlights its relevance in cancer therapeutics.
Industrial Applications
This compound is also explored for its role in developing new materials and chemical processes within the industry. Its unique properties make it suitable for various applications, including polymer synthesis and as a solvent in chemical reactions .
Mechanism of Action
The mechanism of action of N,N-Dimethyl-3-phenylpropanamide involves its interaction with specific molecular targets. The amide group can form hydrogen bonds with proteins and enzymes, influencing their structure and function. The compound may also participate in various biochemical pathways, affecting cellular processes .
Comparison with Similar Compounds
Comparison with Structural Analogs
Table 1: Comparison of N,N-Dialkyl-3-phenylpropanamide Derivatives
Key Observations:
Alkyl Chain Length :
- Dimethyl vs. Diethyl : Dimethyl derivatives are synthesized in higher yields (quantitative vs. 82% for diethyl), likely due to reduced steric hindrance during purification.
- Bulkier Substituents : N,N-Diisopropyl and dibutyl analogs (e.g., CAS 124937-97-9) exhibit increased molecular weight and lipophilicity, impacting solubility and reactivity in catalytic systems.
Reactivity in C–N Bond Cleavage: this compound shows 12% esterification yield under Mn-catalyzed conditions, significantly lower than aromatic amides (e.g., 86% for 4-cyano-N,N-dimethylbenzamide). This highlights the reduced electrophilicity of aliphatic amides compared to aromatic counterparts.
Functional Group Modifications
Aromatic Substitutions:
- Contrastingly, this compound lacks such activating groups, limiting its utility in polymerization.
Amino Derivatives:
- 3-Amino-N,N-dimethyl-3-phenylpropanamide (CAS: 1447447-10-0): The addition of an amino group introduces hydrogen-bonding capacity, making it a candidate for enzyme inhibition studies.
Research Findings and Trends
Catalytic Applications :
- This compound derivatives are critical in asymmetric catalysis. For example, (2R,2'R)-2,2'-((2-iodo-1,3-phenylene)bis(oxy))bis(this compound) facilitates enantioselective fluorochromane synthesis via iodine catalysis.
Pharmaceutical Development: Fragment-based drug discovery utilizes derivatives like (S)-N-(1-(dimethylamino)-1-oxo-3-phenylpropan-2-yl)-4-hydroxyquinoline-6-carboxamide for targeting Factor XI.
Steric and Electronic Effects: Aliphatic substituents reduce reactivity in esterification, while electron-withdrawing groups (e.g., cyano in 4-cyano-N,N-dimethylbenzamide) enhance it.
Biological Activity
N,N-Dimethyl-3-phenylpropanamide (DM-3-PPA) is an organic compound that has garnered interest in medicinal chemistry due to its potential biological activities, particularly in the realm of pain management and receptor modulation. This article explores its biological activity, synthesis, and relevant research findings.
Chemical Structure and Properties
This compound has the molecular formula and a molecular weight of approximately 179.25 g/mol. The compound features a propanamide backbone, which is significant for its interactions with biological targets.
Preliminary studies indicate that DM-3-PPA may modulate the activity of transient receptor potential vanilloid 1 (TRPV1), a receptor involved in pain perception. The interaction with TRPV1 suggests that DM-3-PPA could serve as a potential analgesic agent, possibly offering an alternative to conventional pain relief methods .
Analgesic Potential
Research indicates that DM-3-PPA exhibits notable analgesic properties. In vitro studies have shown that it can influence pain pathways by interacting with TRPV1, which is crucial for the sensation of pain. This interaction could lead to reduced pain perception, making it a candidate for further investigation in pain management therapies .
Binding Affinity and Selectivity
Molecular docking simulations have been employed to predict how DM-3-PPA interacts with TRPV1 and other ion channels. These studies reveal insights into its pharmacodynamics and potential side effects, emphasizing the importance of understanding its binding affinity compared to structurally similar compounds .
Comparative Analysis with Similar Compounds
To better understand the unique properties of DM-3-PPA, a comparison with structurally similar compounds can be insightful:
Compound Name | Structural Feature | Unique Characteristics |
---|---|---|
3-(4-Fluorophenyl)-N,N-dimethylpropanamide | Para-fluorination | May have different receptor binding profiles |
This compound | No fluorine | Serves as a reference for biological activity |
3-(2-Methoxyphenyl)-N,N-dimethylpropanamide | Methoxy substitution | Alters hydrophobicity and electronic effects |
The presence of functional groups significantly influences the biological activity and pharmacological profile of these compounds, highlighting the importance of structural modifications in drug design .
Case Studies and Research Findings
Several studies have explored the synthesis and biological evaluation of DM-3-PPA:
- Synthesis : Various synthetic routes have been developed for DM-3-PPA, typically involving the reaction of benzylamine derivatives with acetamides under specific conditions. Optimizing reaction parameters such as solvent choice and base concentration has been crucial for enhancing yield .
- Biological Evaluation : In vivo studies have demonstrated the analgesic effects of DM-3-PPA in rodent models. These studies often utilize formalin tests to assess pain response, providing quantitative data on its efficacy compared to standard analgesics like morphine .
Q & A
Basic Research Questions
Q. What are the key challenges in synthesizing N,N-Dimethyl-3-phenylpropanamide, and how can reaction conditions be optimized to improve yield?
- Answer : The compound’s synthesis is hindered by its α-proton reactivity and steric hindrance from the dimethylamide group. Evidence from Miyoshi et al. (2020) suggests that temperature control (−40°C), stoichiometric precision (3.0 equivalents of aryl halide), and activating agents (e.g., diiodomethane) are critical for suppressing undesired diarylation . Methodological optimization should involve systematic screening of these parameters using kinetic studies and real-time monitoring (e.g., TLC or HPLC).
Q. Which analytical techniques are most reliable for characterizing this compound and its derivatives?
- Answer : Nuclear Magnetic Resonance (NMR) spectroscopy (¹H, ¹³C) is essential for confirming regiochemistry and purity, as demonstrated in the synthesis of fluorinated derivatives . High-resolution mass spectrometry (HRMS) and chromatography (GC/HPLC) with standardized reference materials (e.g., USP guidelines for dimethylamide solvents ) ensure reproducibility. For intermediates, X-ray crystallography can resolve stereochemical ambiguities .
Q. How do solvent polarity and temperature influence the chemoselectivity of this compound in alkylation reactions?
- Answer : Polar aprotic solvents (e.g., DMF) enhance nucleophilicity but may promote side reactions. Strontium-mediated alkylation at −40°C in THF suppresses competing pathways, achieving >75% yield for monophenylated ketones . Solvent effects should be modeled using computational tools (e.g., COSMO-RS) alongside empirical testing.
Advanced Research Questions
Q. How does stereochemistry impact the pharmacological activity of this compound derivatives?
- Answer : Stereoisomers of related compounds (e.g., ohmefentanyl analogs) show >10,000-fold differences in opioid receptor affinity . For this compound derivatives, enantioselective synthesis (e.g., iodine(I/III) catalysis ) and chiral resolution (HPLC with amylose columns) are critical. Activity assays (GPI/MVD contraction inhibition) paired with molecular docking can map stereochemical contributions to receptor binding .
Q. What strategies resolve contradictions in reported bioactivity data for this compound analogs?
- Answer : Apply meta-analysis frameworks (e.g., Cochrane guidelines ) to assess heterogeneity. Use Higgins’ I² statistic to quantify variability across studies; values >50% indicate significant heterogeneity requiring subgroup analysis (e.g., by assay type or cell line) . Validate findings using orthogonal assays (e.g., radioligand binding vs. functional GPI/MVD tests) .
Q. How can fragment-based lead generation (FBLG) improve the design of this compound-derived inhibitors?
- Answer : Fragment libraries targeting the compound’s amide core (e.g., 4-hydroxyquinoline hybrids ) enable structure-activity relationship (SAR) studies. Use surface plasmon resonance (SPR) or microscale thermophoresis (MST) to screen fragments for binding affinity. Optimize hits via iterative medicinal chemistry cycles, prioritizing substituents that enhance selectivity (e.g., para-fluoro groups to reduce off-target effects) .
Q. What mechanistic insights explain the compound’s role in catalytic C–H activation reactions?
- Answer : The dimethylamide group acts as a directing group, facilitating metal insertion into proximal C–H bonds. Studies on strontium-mediated alkylation suggest a radical-intermediate pathway, supported by ESR spectroscopy . Computational studies (DFT) can model transition states and guide ligand design to improve catalytic turnover.
Q. Methodological Guidance Tables
Table 1. Key Parameters for Optimizing Alkylation Reactions
Parameter | Optimal Range | Impact on Yield/Selectivity | Reference |
---|---|---|---|
Temperature | −40°C to −30°C | Suppresses diarylation | |
Activating Agent | 0.1 eq. CH₂I₂ | Enhances Sr reactivity | |
Solvent | THF | Balances polarity and stability |
Table 2. Statistical Tools for Resolving Data Contradictions
Properties
IUPAC Name |
N,N-dimethyl-3-phenylpropanamide | |
---|---|---|
Source | PubChem | |
URL | https://pubchem.ncbi.nlm.nih.gov | |
Description | Data deposited in or computed by PubChem | |
InChI |
InChI=1S/C11H15NO/c1-12(2)11(13)9-8-10-6-4-3-5-7-10/h3-7H,8-9H2,1-2H3 | |
Source | PubChem | |
URL | https://pubchem.ncbi.nlm.nih.gov | |
Description | Data deposited in or computed by PubChem | |
InChI Key |
FDBVFKPSUNQAHK-UHFFFAOYSA-N | |
Source | PubChem | |
URL | https://pubchem.ncbi.nlm.nih.gov | |
Description | Data deposited in or computed by PubChem | |
Canonical SMILES |
CN(C)C(=O)CCC1=CC=CC=C1 | |
Source | PubChem | |
URL | https://pubchem.ncbi.nlm.nih.gov | |
Description | Data deposited in or computed by PubChem | |
Molecular Formula |
C11H15NO | |
Source | PubChem | |
URL | https://pubchem.ncbi.nlm.nih.gov | |
Description | Data deposited in or computed by PubChem | |
DSSTOX Substance ID |
DTXSID10207028 | |
Record name | N,N-Dimethylhydrocinnamide | |
Source | EPA DSSTox | |
URL | https://comptox.epa.gov/dashboard/DTXSID10207028 | |
Description | DSSTox provides a high quality public chemistry resource for supporting improved predictive toxicology. | |
Molecular Weight |
177.24 g/mol | |
Source | PubChem | |
URL | https://pubchem.ncbi.nlm.nih.gov | |
Description | Data deposited in or computed by PubChem | |
CAS No. |
5830-31-9 | |
Record name | N,N-Dimethylhydrocinnamide | |
Source | ChemIDplus | |
URL | https://pubchem.ncbi.nlm.nih.gov/substance/?source=chemidplus&sourceid=0005830319 | |
Description | ChemIDplus is a free, web search system that provides access to the structure and nomenclature authority files used for the identification of chemical substances cited in National Library of Medicine (NLM) databases, including the TOXNET system. | |
Record name | N,N-Dimethylhydrocinnamide | |
Source | EPA DSSTox | |
URL | https://comptox.epa.gov/dashboard/DTXSID10207028 | |
Description | DSSTox provides a high quality public chemistry resource for supporting improved predictive toxicology. | |
Retrosynthesis Analysis
AI-Powered Synthesis Planning: Our tool employs the Template_relevance Pistachio, Template_relevance Bkms_metabolic, Template_relevance Pistachio_ringbreaker, Template_relevance Reaxys, Template_relevance Reaxys_biocatalysis model, leveraging a vast database of chemical reactions to predict feasible synthetic routes.
One-Step Synthesis Focus: Specifically designed for one-step synthesis, it provides concise and direct routes for your target compounds, streamlining the synthesis process.
Accurate Predictions: Utilizing the extensive PISTACHIO, BKMS_METABOLIC, PISTACHIO_RINGBREAKER, REAXYS, REAXYS_BIOCATALYSIS database, our tool offers high-accuracy predictions, reflecting the latest in chemical research and data.
Strategy Settings
Precursor scoring | Relevance Heuristic |
---|---|
Min. plausibility | 0.01 |
Model | Template_relevance |
Template Set | Pistachio/Bkms_metabolic/Pistachio_ringbreaker/Reaxys/Reaxys_biocatalysis |
Top-N result to add to graph | 6 |
Feasible Synthetic Routes
Disclaimer and Information on In-Vitro Research Products
Please be aware that all articles and product information presented on BenchChem are intended solely for informational purposes. The products available for purchase on BenchChem are specifically designed for in-vitro studies, which are conducted outside of living organisms. In-vitro studies, derived from the Latin term "in glass," involve experiments performed in controlled laboratory settings using cells or tissues. It is important to note that these products are not categorized as medicines or drugs, and they have not received approval from the FDA for the prevention, treatment, or cure of any medical condition, ailment, or disease. We must emphasize that any form of bodily introduction of these products into humans or animals is strictly prohibited by law. It is essential to adhere to these guidelines to ensure compliance with legal and ethical standards in research and experimentation.