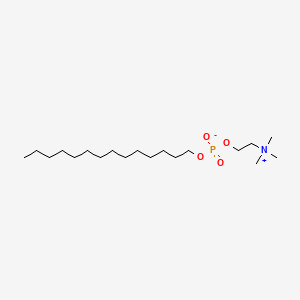
Tetradecylphosphocholine
Overview
Description
Tetradecylphosphocholine is a zwitterionic surfactant commonly used in the solubilization, stabilization, and purification of membrane proteins. It belongs to the class of phosphocholines, which are known for their ability to mimic the properties of lipid bilayers, making them valuable in various biochemical and biophysical studies .
Preparation Methods
Synthetic Routes and Reaction Conditions: Tetradecylphosphocholine can be synthesized through a series of chemical reactions involving the alkylation of phosphocholine with tetradecyl halides. The reaction typically requires a base such as sodium hydroxide or potassium carbonate to facilitate the nucleophilic substitution reaction. The process involves heating the reactants under reflux conditions to ensure complete conversion .
Industrial Production Methods: In industrial settings, this compound is produced using large-scale reactors where the reactants are mixed and heated under controlled conditions. The product is then purified through crystallization or chromatography to achieve high purity levels suitable for scientific research .
Chemical Reactions Analysis
Types of Reactions: Tetradecylphosphocholine primarily undergoes substitution reactions due to the presence of the phosphocholine group. It can also participate in oxidation and reduction reactions under specific conditions .
Common Reagents and Conditions:
Substitution Reactions: Typically involve alkyl halides and a base such as sodium hydroxide.
Oxidation Reactions: Can be carried out using oxidizing agents like hydrogen peroxide or potassium permanganate.
Reduction Reactions: Often involve reducing agents such as sodium borohydride or lithium aluminum hydride.
Major Products: The major products formed from these reactions depend on the specific reagents and conditions used. For example, substitution reactions with alkyl halides yield alkylated phosphocholine derivatives, while oxidation reactions can produce phosphocholine oxides .
Scientific Research Applications
Tetradecylphosphocholine has a wide range of applications in scientific research:
Mechanism of Action
Tetradecylphosphocholine exerts its effects by interacting with the lipid bilayers of cell membranes. It integrates into the membrane, disrupting the lipid structure and increasing membrane fluidity. This action facilitates the solubilization of membrane proteins, making them accessible for further study. The compound targets membrane proteins and pathways involved in cellular signaling and transport .
Comparison with Similar Compounds
Dodecylphosphocholine: Another phosphocholine with a shorter alkyl chain, used for similar applications but with different solubilization properties.
Hexadecylphosphocholine: A phosphocholine with a longer alkyl chain, offering different stability and solubilization characteristics.
Uniqueness: Tetradecylphosphocholine is unique due to its optimal chain length, which provides a balance between solubilization efficiency and membrane stability. This makes it particularly effective for the solubilization and stabilization of a wide range of membrane proteins .
Biological Activity
Tetradecylphosphocholine (TPC), also known as Fos-choline-14, is a synthetic zwitterionic phospholipid analog primarily used in research as a detergent for membrane protein solubilization. Its biological activity has been extensively studied, revealing various effects on cellular processes, antimicrobial properties, and potential therapeutic applications.
Chemical Structure and Properties
TPC is characterized by a long hydrophobic tetradecyl chain and a phosphocholine head group, which imparts unique amphiphilic properties. This structure allows TPC to interact with biological membranes, making it an effective tool for studying membrane proteins.
1. Membrane Protein Solubilization
TPC is widely utilized in biochemistry for the solubilization of membrane proteins. Its effectiveness as a detergent stems from its ability to disrupt lipid bilayers while maintaining protein functionality. Research indicates that TPC can stabilize proteins in solution, facilitating structural and functional studies of membrane proteins .
2. Antimicrobial Properties
Recent studies have highlighted the antimicrobial potential of TPC. It has shown efficacy against various bacterial strains, likely due to its ability to disrupt microbial membranes. For instance, TPC demonstrated bactericidal activity comparable to other alkyl phosphocholines, suggesting its potential use in developing antimicrobial agents .
3. Inhibition of Phosphatidylcholine Biosynthesis
Research has indicated that TPC can inhibit phosphatidylcholine biosynthesis, which is crucial for maintaining cell membrane integrity. This inhibition may have implications for cancer therapy, as altered lipid metabolism is often associated with tumorigenesis .
Case Study 1: Antimicrobial Efficacy
A study evaluated the antimicrobial activity of TPC against Staphylococcus aureus. Results showed that TPC effectively inhibited bacterial growth at concentrations as low as 0.5% (v/v), demonstrating its potential as an antimicrobial agent in clinical settings .
Case Study 2: Membrane Protein Research
In a study focusing on mitochondrial carriers, TPC was used to solubilize the mitochondrial ATP-binding cassette transporter Mdl1. The research revealed that TPC not only preserved the functional state of Mdl1 but also allowed for detailed structural analysis through NMR spectroscopy .
Research Findings
Properties
IUPAC Name |
tetradecyl 2-(trimethylazaniumyl)ethyl phosphate | |
---|---|---|
Source | PubChem | |
URL | https://pubchem.ncbi.nlm.nih.gov | |
Description | Data deposited in or computed by PubChem | |
InChI |
InChI=1S/C19H42NO4P/c1-5-6-7-8-9-10-11-12-13-14-15-16-18-23-25(21,22)24-19-17-20(2,3)4/h5-19H2,1-4H3 | |
Source | PubChem | |
URL | https://pubchem.ncbi.nlm.nih.gov | |
Description | Data deposited in or computed by PubChem | |
InChI Key |
BETUMLXGYDBOLV-UHFFFAOYSA-N | |
Source | PubChem | |
URL | https://pubchem.ncbi.nlm.nih.gov | |
Description | Data deposited in or computed by PubChem | |
Canonical SMILES |
CCCCCCCCCCCCCCOP(=O)([O-])OCC[N+](C)(C)C | |
Source | PubChem | |
URL | https://pubchem.ncbi.nlm.nih.gov | |
Description | Data deposited in or computed by PubChem | |
Molecular Formula |
C19H42NO4P | |
Source | PubChem | |
URL | https://pubchem.ncbi.nlm.nih.gov | |
Description | Data deposited in or computed by PubChem | |
DSSTOX Substance ID |
DTXSID50228358 | |
Record name | N-Tetradecylphosphorylcholine | |
Source | EPA DSSTox | |
URL | https://comptox.epa.gov/dashboard/DTXSID50228358 | |
Description | DSSTox provides a high quality public chemistry resource for supporting improved predictive toxicology. | |
Molecular Weight |
379.5 g/mol | |
Source | PubChem | |
URL | https://pubchem.ncbi.nlm.nih.gov | |
Description | Data deposited in or computed by PubChem | |
CAS No. |
77733-28-9 | |
Record name | N-Tetradecylphosphorylcholine | |
Source | ChemIDplus | |
URL | https://pubchem.ncbi.nlm.nih.gov/substance/?source=chemidplus&sourceid=0077733289 | |
Description | ChemIDplus is a free, web search system that provides access to the structure and nomenclature authority files used for the identification of chemical substances cited in National Library of Medicine (NLM) databases, including the TOXNET system. | |
Record name | N-Tetradecylphosphorylcholine | |
Source | EPA DSSTox | |
URL | https://comptox.epa.gov/dashboard/DTXSID50228358 | |
Description | DSSTox provides a high quality public chemistry resource for supporting improved predictive toxicology. | |
Retrosynthesis Analysis
AI-Powered Synthesis Planning: Our tool employs the Template_relevance Pistachio, Template_relevance Bkms_metabolic, Template_relevance Pistachio_ringbreaker, Template_relevance Reaxys, Template_relevance Reaxys_biocatalysis model, leveraging a vast database of chemical reactions to predict feasible synthetic routes.
One-Step Synthesis Focus: Specifically designed for one-step synthesis, it provides concise and direct routes for your target compounds, streamlining the synthesis process.
Accurate Predictions: Utilizing the extensive PISTACHIO, BKMS_METABOLIC, PISTACHIO_RINGBREAKER, REAXYS, REAXYS_BIOCATALYSIS database, our tool offers high-accuracy predictions, reflecting the latest in chemical research and data.
Strategy Settings
Precursor scoring | Relevance Heuristic |
---|---|
Min. plausibility | 0.01 |
Model | Template_relevance |
Template Set | Pistachio/Bkms_metabolic/Pistachio_ringbreaker/Reaxys/Reaxys_biocatalysis |
Top-N result to add to graph | 6 |
Feasible Synthetic Routes
Disclaimer and Information on In-Vitro Research Products
Please be aware that all articles and product information presented on BenchChem are intended solely for informational purposes. The products available for purchase on BenchChem are specifically designed for in-vitro studies, which are conducted outside of living organisms. In-vitro studies, derived from the Latin term "in glass," involve experiments performed in controlled laboratory settings using cells or tissues. It is important to note that these products are not categorized as medicines or drugs, and they have not received approval from the FDA for the prevention, treatment, or cure of any medical condition, ailment, or disease. We must emphasize that any form of bodily introduction of these products into humans or animals is strictly prohibited by law. It is essential to adhere to these guidelines to ensure compliance with legal and ethical standards in research and experimentation.