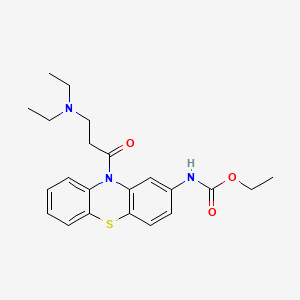
Ethacizine
Overview
Description
Preparation Methods
Synthetic Routes and Reaction Conditions
The synthesis of NIK-244 involves the reaction of 10-(3-chloropropionyl)-2-(ethoxycarbonylamino)phenothiazine with diethylamine in refluxing toluene . This reaction results in the formation of Ethacizine hydrochloride.
Industrial Production Methods
Industrial production methods for NIK-244 are not extensively documented in the available literature. the synthesis typically involves standard organic synthesis techniques, including the use of appropriate solvents, reagents, and reaction conditions to ensure high yield and purity.
Chemical Reactions Analysis
Types of Reactions
NIK-244 undergoes various chemical reactions, including:
Oxidation: This reaction involves the addition of oxygen or the removal of hydrogen.
Reduction: This reaction involves the addition of hydrogen or the removal of oxygen.
Substitution: This reaction involves the replacement of one atom or group of atoms with another.
Common Reagents and Conditions
Common reagents used in the reactions involving NIK-244 include oxidizing agents, reducing agents, and various nucleophiles for substitution reactions. The specific conditions depend on the desired reaction and product.
Major Products Formed
The major products formed from these reactions depend on the type of reaction and the reagents used. For example, oxidation reactions may produce oxidized derivatives, while substitution reactions may result in various substituted products.
Scientific Research Applications
Pharmacological Properties
Ethacizine exhibits significant antiarrhythmic effects, particularly in patients with coronary artery disease. It has been shown to prolong the refractory period of cardiac tissues, thereby reducing the frequency of arrhythmias. The compound's mechanism of action involves blocking sodium channels, which stabilizes cardiac membranes and prevents abnormal electrical conduction.
Clinical Applications
-
Management of Ventricular Tachycardia :
- This compound has been evaluated for its effectiveness in treating sustained ventricular tachycardia (VT) in patients with coronary artery disease. A study indicated that it was effective in preventing recurrences of VT in most patients when administered long-term at doses ranging from 200 to 400 mg per day .
-
Treatment of Supraventricular Tachycardia :
- In a comparative study involving patients with chronic ventricular rhythm disorders, this compound demonstrated an antiarrhythmic effect in 81% of cases, outperforming other agents like Ritmilen and etmozin . This highlights its utility in managing conditions such as atrial fibrillation and frequent premature atrial contractions.
- Antianginal Properties :
- Case Studies :
Efficacy and Safety
This compound's efficacy has been consistently reported across various studies. In a cohort study involving 56 patients with paroxysmal atrial fibrillation, this compound successfully removed and prevented episodes in 60-65% of cases . However, it is important to note that some patients experienced side effects such as prolonged P-Q intervals and widened QRS complexes on ECG, indicating the need for careful monitoring during treatment .
Comparative Studies
A comparative analysis between this compound and other antiarrhythmic agents revealed that while this compound had a higher efficacy rate, other medications like Ritmilen showed lower effectiveness coupled with higher incidences of side effects . This positions this compound as a favorable option for patients with severe arrhythmias who do not respond to first-line treatments.
Summary Table of Clinical Findings
Mechanism of Action
NIK-244 exerts its effects by inhibiting the depolarizing current responsible for intraatrial and His-Purkinje-ventricular conduction . This inhibition results in the stabilization of cardiac electrical activity, thereby preventing irregular heartbeats. The molecular targets include voltage-gated sodium channels, which are crucial for the propagation of electrical signals in the heart .
Comparison with Similar Compounds
Similar Compounds
Flecainide: Another Class Ic antiarrhythmic agent with a similar mechanism of action but shorter duration of effect compared to NIK-244.
Propafenone: A Class Ic antiarrhythmic agent with similar properties but different pharmacokinetics.
Uniqueness of NIK-244
NIK-244 is unique due to its longer-lasting antiarrhythmic effects compared to other Class Ic agents like Flecainide . This makes it a valuable compound for the treatment of arrhythmias, providing prolonged stabilization of cardiac electrical activity.
Biological Activity
Ethacizine, also known as ethacyzine, is a class Ic antiarrhythmic agent primarily used for treating severe ventricular and supraventricular arrhythmias. It is particularly effective in patients with organic heart disease and those experiencing refractory tachycardia associated with Wolff–Parkinson–White syndrome. The compound has been utilized mainly in Russia and other CIS countries, with its therapeutic efficacy and safety profile being subjects of various clinical studies.
- IUPAC Name : Not specified in the sources.
- Molecular Formula : CHNOS
- Molar Mass : 413.54 g/mol
- Bioavailability : Approximately 40% when administered orally.
- Protein Binding : 90%
- Elimination Half-life : 2.5 hours
This compound works by blocking sodium channels, thereby stabilizing the cardiac membrane and reducing excitability. This action helps to control abnormal heart rhythms effectively.
Case Studies and Research Findings
-
Efficacy in Atrial and Ventricular Arrhythmias
- A prospective study involving 98 patients demonstrated that this compound (50 mg twice daily) significantly reduced premature atrial contractions (PACs) and premature ventricular contractions (PVCs). The study reported an 84.8% reduction in PVCs over a follow-up period of 150-210 days, with adverse effects noted in only 4% of subjects .
-
Long-term Use and Safety
- A retrospective analysis over 30 years showed that this compound was effective in 71.3% of patients treated for arrhythmias, including a success rate of 61.7% for atrial fibrillation (AF) interruption and 78.9% for controlling ventricular ectopy . Importantly, no serious adverse events were recorded during long-term use, indicating a favorable safety profile.
- Acute Myocardial Infarction
Summary of Clinical Findings
Study Type | Population Size | Efficacy Rate | Notable Findings |
---|---|---|---|
Prospective Study | 98 | 84.8% PVC reduction | Minimal adverse effects (4%) |
Retrospective Study | 74 | 71.3% overall | Long-term safety with no serious events |
Acute Care | Varies | 82.4% | Effective in acute myocardial infarction cases |
Adverse Effects
While this compound is generally well-tolerated, some reported adverse effects include:
- Second-degree AV block (Mobitz type I)
- Sustained ventricular tachycardia
- Asymptomatic nonsustained polytopic ventricular tachycardia
- Transient ST-segment elevation observed via Holter monitoring .
These effects are typically manageable and occur infrequently, reinforcing the drug's overall safety when monitored appropriately.
Properties
IUPAC Name |
ethyl N-[10-[3-(diethylamino)propanoyl]phenothiazin-2-yl]carbamate | |
---|---|---|
Source | PubChem | |
URL | https://pubchem.ncbi.nlm.nih.gov | |
Description | Data deposited in or computed by PubChem | |
InChI |
InChI=1S/C22H27N3O3S/c1-4-24(5-2)14-13-21(26)25-17-9-7-8-10-19(17)29-20-12-11-16(15-18(20)25)23-22(27)28-6-3/h7-12,15H,4-6,13-14H2,1-3H3,(H,23,27) | |
Source | PubChem | |
URL | https://pubchem.ncbi.nlm.nih.gov | |
Description | Data deposited in or computed by PubChem | |
InChI Key |
PQXGNJKJMFUPPM-UHFFFAOYSA-N | |
Source | PubChem | |
URL | https://pubchem.ncbi.nlm.nih.gov | |
Description | Data deposited in or computed by PubChem | |
Canonical SMILES |
CCN(CC)CCC(=O)N1C2=CC=CC=C2SC3=C1C=C(C=C3)NC(=O)OCC | |
Source | PubChem | |
URL | https://pubchem.ncbi.nlm.nih.gov | |
Description | Data deposited in or computed by PubChem | |
Molecular Formula |
C22H27N3O3S | |
Source | PubChem | |
URL | https://pubchem.ncbi.nlm.nih.gov | |
Description | Data deposited in or computed by PubChem | |
DSSTOX Substance ID |
DTXSID50187057 | |
Record name | Ethacizine | |
Source | EPA DSSTox | |
URL | https://comptox.epa.gov/dashboard/DTXSID50187057 | |
Description | DSSTox provides a high quality public chemistry resource for supporting improved predictive toxicology. | |
Molecular Weight |
413.5 g/mol | |
Source | PubChem | |
URL | https://pubchem.ncbi.nlm.nih.gov | |
Description | Data deposited in or computed by PubChem | |
CAS No. |
33414-33-4 | |
Record name | Ethyl N-[10-[3-(diethylamino)-1-oxopropyl]-10H-phenothiazin-2-yl]carbamate | |
Source | CAS Common Chemistry | |
URL | https://commonchemistry.cas.org/detail?cas_rn=33414-33-4 | |
Description | CAS Common Chemistry is an open community resource for accessing chemical information. Nearly 500,000 chemical substances from CAS REGISTRY cover areas of community interest, including common and frequently regulated chemicals, and those relevant to high school and undergraduate chemistry classes. This chemical information, curated by our expert scientists, is provided in alignment with our mission as a division of the American Chemical Society. | |
Explanation | The data from CAS Common Chemistry is provided under a CC-BY-NC 4.0 license, unless otherwise stated. | |
Record name | Ethacizine | |
Source | ChemIDplus | |
URL | https://pubchem.ncbi.nlm.nih.gov/substance/?source=chemidplus&sourceid=0033414334 | |
Description | ChemIDplus is a free, web search system that provides access to the structure and nomenclature authority files used for the identification of chemical substances cited in National Library of Medicine (NLM) databases, including the TOXNET system. | |
Record name | Ethacizine | |
Source | DrugBank | |
URL | https://www.drugbank.ca/drugs/DB13645 | |
Description | The DrugBank database is a unique bioinformatics and cheminformatics resource that combines detailed drug (i.e. chemical, pharmacological and pharmaceutical) data with comprehensive drug target (i.e. sequence, structure, and pathway) information. | |
Explanation | Creative Common's Attribution-NonCommercial 4.0 International License (http://creativecommons.org/licenses/by-nc/4.0/legalcode) | |
Record name | Ethacizin | |
Source | DTP/NCI | |
URL | https://dtp.cancer.gov/dtpstandard/servlet/dwindex?searchtype=NSC&outputformat=html&searchlist=621878 | |
Description | The NCI Development Therapeutics Program (DTP) provides services and resources to the academic and private-sector research communities worldwide to facilitate the discovery and development of new cancer therapeutic agents. | |
Explanation | Unless otherwise indicated, all text within NCI products is free of copyright and may be reused without our permission. Credit the National Cancer Institute as the source. | |
Record name | Ethacizine | |
Source | EPA DSSTox | |
URL | https://comptox.epa.gov/dashboard/DTXSID50187057 | |
Description | DSSTox provides a high quality public chemistry resource for supporting improved predictive toxicology. | |
Record name | ETHACIZINE | |
Source | FDA Global Substance Registration System (GSRS) | |
URL | https://gsrs.ncats.nih.gov/ginas/app/beta/substances/FE5SPV1Z6G | |
Description | The FDA Global Substance Registration System (GSRS) enables the efficient and accurate exchange of information on what substances are in regulated products. Instead of relying on names, which vary across regulatory domains, countries, and regions, the GSRS knowledge base makes it possible for substances to be defined by standardized, scientific descriptions. | |
Explanation | Unless otherwise noted, the contents of the FDA website (www.fda.gov), both text and graphics, are not copyrighted. They are in the public domain and may be republished, reprinted and otherwise used freely by anyone without the need to obtain permission from FDA. Credit to the U.S. Food and Drug Administration as the source is appreciated but not required. | |
Retrosynthesis Analysis
AI-Powered Synthesis Planning: Our tool employs the Template_relevance Pistachio, Template_relevance Bkms_metabolic, Template_relevance Pistachio_ringbreaker, Template_relevance Reaxys, Template_relevance Reaxys_biocatalysis model, leveraging a vast database of chemical reactions to predict feasible synthetic routes.
One-Step Synthesis Focus: Specifically designed for one-step synthesis, it provides concise and direct routes for your target compounds, streamlining the synthesis process.
Accurate Predictions: Utilizing the extensive PISTACHIO, BKMS_METABOLIC, PISTACHIO_RINGBREAKER, REAXYS, REAXYS_BIOCATALYSIS database, our tool offers high-accuracy predictions, reflecting the latest in chemical research and data.
Strategy Settings
Precursor scoring | Relevance Heuristic |
---|---|
Min. plausibility | 0.01 |
Model | Template_relevance |
Template Set | Pistachio/Bkms_metabolic/Pistachio_ringbreaker/Reaxys/Reaxys_biocatalysis |
Top-N result to add to graph | 6 |
Feasible Synthetic Routes
Q1: How does ethacizine exert its antiarrhythmic effects?
A1: this compound primarily acts by blocking fast sodium channels in the heart muscle. [] This blockade slows down the rapid depolarization phase of the cardiac action potential, thereby reducing the conduction velocity of electrical impulses within the heart. [, , ]
Q2: What are the downstream consequences of this compound's sodium channel blocking activity?
A2: By slowing down conduction, this compound:
- Increases the effective refractory period of cardiac cells: This reduces the excitability of the heart muscle, making it less likely to generate abnormal rhythms. []
- Suppresses abnormal automaticity: It reduces the spontaneous firing rate of cells that can trigger arrhythmias. []
- Prolongs the QRS complex on an electrocardiogram (ECG): This reflects the slowed conduction through the ventricles. [, ]
Q3: Does this compound interact with other ion channels or receptors?
A3: Research suggests that this compound also interacts with:
- Calcium channels: It inhibits slow calcium channels, particularly at higher concentrations, contributing to its negative inotropic effect (reduced force of contraction). [, ]
- Muscarinic cholinergic receptors: It shows affinity for muscarinic receptors, particularly the M2 subtype, suggesting a potential role in its antiarrhythmic action. []
- Adrenergic receptors: this compound exhibits weak affinity for alpha-adrenergic receptors but does not significantly affect beta-adrenergic receptors. []
Q4: What is the chemical structure of this compound?
A4: this compound is a phenothiazine derivative. Its chemical structure consists of a phenothiazine ring system substituted with a carbethoxyamino group at position 2 and a diethylaminopropionyl group at position 10.
Q5: What is the molecular formula and weight of this compound?
A5: this compound has the molecular formula C20H25N3O2S•HCl and a molecular weight of 407.97 g/mol.
Q6: Is there any spectroscopic data available for this compound?
A6: While the provided research does not delve into detailed spectroscopic analysis, standard techniques like NMR, IR, and mass spectrometry can be employed for structural characterization.
Q7: Are there any studies on the material compatibility and stability of this compound?
A7: The provided research primarily focuses on the pharmacological aspects of this compound. Further investigation is needed to determine its compatibility with various materials and its stability under different conditions.
Q8: Does this compound possess any catalytic properties?
A8: this compound is primarily recognized for its antiarrhythmic activity. Based on its structure and known mechanisms, it is unlikely to possess significant catalytic properties.
Q9: Have computational methods been used to study this compound?
A9: While the research provided does not explicitly mention computational studies on this compound, computational chemistry techniques like molecular docking and QSAR modeling could be employed to further investigate its interactions with target proteins and explore structure-activity relationships. []
Q10: How do structural modifications affect the activity of this compound?
A10: Structural modifications, particularly in the side chain at the nitrogen atom in position 10 of the phenothiazine ring, can influence this compound's antiarrhythmic activity and toxicity. [] For example, replacing the diethylamine group with a demethylamine group increases toxicity while enhancing antiarrhythmic effects. Lengthening the side chain also impacts activity.
Q11: What are the SHE (Safety, Health, and Environment) considerations for this compound?
A11: As with any pharmaceutical compound, handling this compound requires adherence to standard SHE regulations. This includes appropriate personal protective equipment, safe handling practices, and proper waste disposal procedures. Further research may be needed to assess its environmental impact and degradation pathways fully.
Q12: How is this compound absorbed, distributed, metabolized, and excreted (ADME)?
A12: * Absorption: this compound is well-absorbed after oral administration, but its bioavailability is lower in patients with myocardial infarction. [, ]* Distribution: It distributes widely in the body.* Metabolism: this compound undergoes hepatic metabolism.* Excretion: It is primarily excreted in the urine.
Q13: Are there any differences in the pharmacokinetics of this compound in different patient populations?
A13: Yes, research indicates that patients with myocardial infarction exhibit a lower bioavailability of this compound compared to those with other conditions. [, ] This difference highlights the importance of considering individual patient factors when optimizing therapy.
Q14: How does the pharmacokinetic profile of this compound relate to its dosing regimen?
A14: While specific dosing information is outside the scope of this scientific Q&A, the pharmacokinetic properties of this compound, including its absorption, elimination half-life, and potential drug interactions, are crucial factors in determining appropriate dosage regimens.
Q15: What in vitro and in vivo models have been used to study the efficacy of this compound?
A15: this compound's efficacy has been investigated using various models, including:
- Cellular models: Studies on isolated cardiac myocytes have been conducted to examine its effects on action potentials, ion currents, and contractile force. [, ]
- Animal models: Research involving animal models of arrhythmias, such as aconitine-induced arrhythmias in rats and ischemia-induced arrhythmias in dogs and cats, has demonstrated its antiarrhythmic activity. [, , , ]
- Clinical trials: Several clinical trials have assessed the efficacy of this compound in patients with various cardiac arrhythmias, including ventricular tachycardia, supraventricular tachycardia, and atrial fibrillation. [, , , , , , , , , ]
Disclaimer and Information on In-Vitro Research Products
Please be aware that all articles and product information presented on BenchChem are intended solely for informational purposes. The products available for purchase on BenchChem are specifically designed for in-vitro studies, which are conducted outside of living organisms. In-vitro studies, derived from the Latin term "in glass," involve experiments performed in controlled laboratory settings using cells or tissues. It is important to note that these products are not categorized as medicines or drugs, and they have not received approval from the FDA for the prevention, treatment, or cure of any medical condition, ailment, or disease. We must emphasize that any form of bodily introduction of these products into humans or animals is strictly prohibited by law. It is essential to adhere to these guidelines to ensure compliance with legal and ethical standards in research and experimentation.