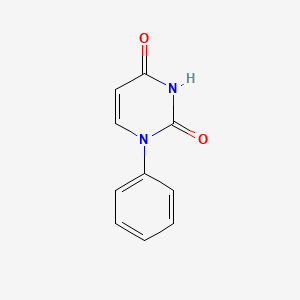
1-Phenyluracil
Overview
Description
1-Phenyluracil is a derivative of uracil, a pyrimidine nucleobase found in RNA. This compound features a phenyl group attached to the nitrogen atom at position 1 of the uracil ring. It is known for its diverse applications in scientific research, particularly in the fields of chemistry, biology, and medicine.
Mechanism of Action
Target of Action
1-Phenyluracil is a pyrimidine analog, similar to Fluorouracil . Pyrimidine analogs typically target enzymes involved in DNA synthesis, such as thymidylate synthase . Thymidylate synthase plays a crucial role in the synthesis of thymidine monophosphate (dTMP), which is essential for DNA replication and repair .
Mode of Action
Based on its structural similarity to fluorouracil, it can be inferred that this compound might also inhibit thymidylate synthase . This inhibition can prevent the conversion of deoxyuridylic acid to thymidylic acid, thereby disrupting DNA synthesis and leading to cell death .
Biochemical Pathways
This compound, being a pyrimidine analog, is likely to affect the pyrimidine biosynthesis pathway. The inhibition of thymidylate synthase disrupts the conversion of deoxyuridine monophosphate (dUMP) to deoxythymidine monophosphate (dTMP). This disruption can lead to an imbalance in the nucleotide pool, causing DNA damage and triggering apoptosis .
Result of Action
The primary result of this compound’s action would be the inhibition of DNA synthesis, leading to cell death. This is due to the disruption of the pyrimidine biosynthesis pathway, causing DNA damage and triggering apoptosis .
Biochemical Analysis
Biochemical Properties
1-Phenyluracil plays a significant role in biochemical reactions due to its ability to interact with various enzymes and proteins. It has been observed to interact with enzymes such as uracil-DNA glycosylase, which is involved in the base excision repair pathway. This interaction is crucial for the removal of uracil from DNA, thereby maintaining DNA integrity . Additionally, this compound can bind to certain proteins, influencing their activity and stability. The nature of these interactions often involves hydrogen bonding and hydrophobic interactions, which stabilize the enzyme-substrate complex.
Cellular Effects
This compound affects various cellular processes, including cell signaling pathways, gene expression, and cellular metabolism. It has been shown to influence the expression of genes involved in DNA repair and replication . In terms of cellular metabolism, this compound can alter the metabolic flux by interacting with key metabolic enzymes, thereby affecting the overall metabolic state of the cell. These effects are critical in understanding how cells respond to DNA damage and repair mechanisms.
Molecular Mechanism
At the molecular level, this compound exerts its effects through specific binding interactions with biomolecules. It can inhibit or activate enzymes by binding to their active sites or allosteric sites. For example, its interaction with uracil-DNA glycosylase involves the formation of a stable enzyme-substrate complex, which facilitates the excision of uracil from DNA . This binding interaction is crucial for the enzyme’s catalytic activity and overall function in DNA repair pathways.
Temporal Effects in Laboratory Settings
In laboratory settings, the effects of this compound can change over time. Studies have shown that the compound is relatively stable under standard laboratory conditions, but it can degrade over extended periods . The long-term effects of this compound on cellular function have been observed in both in vitro and in vivo studies, indicating that prolonged exposure can lead to changes in gene expression and cellular metabolism. These temporal effects are important for understanding the compound’s stability and efficacy in experimental settings.
Dosage Effects in Animal Models
The effects of this compound vary with different dosages in animal models. At low doses, the compound has been shown to enhance DNA repair mechanisms without causing significant toxicity . At higher doses, this compound can exhibit toxic effects, including cellular apoptosis and necrosis. These dosage-dependent effects are crucial for determining the therapeutic window and potential side effects of the compound in preclinical studies.
Metabolic Pathways
This compound is involved in several metabolic pathways, including those related to nucleotide metabolism and DNA repair . It interacts with enzymes such as uracil-DNA glycosylase and other nucleotide-processing enzymes, affecting the levels of various metabolites. These interactions can influence the overall metabolic flux, leading to changes in the cellular metabolic state.
Transport and Distribution
Within cells and tissues, this compound is transported and distributed through passive diffusion and active transport mechanisms . It can interact with transport proteins and binding proteins, which facilitate its movement across cellular membranes. The distribution of this compound is generally uneven, with higher concentrations observed in tissues with high metabolic activity.
Subcellular Localization
This compound is localized in various subcellular compartments, including the nucleus and cytoplasm . Its activity and function can be influenced by its subcellular localization, as it may interact with different biomolecules in distinct compartments. For instance, in the nucleus, this compound can interact with DNA repair enzymes, while in the cytoplasm, it may influence metabolic enzymes. The localization of this compound is often directed by specific targeting signals and post-translational modifications.
Preparation Methods
Synthetic Routes and Reaction Conditions: 1-Phenyluracil can be synthesized through various methods. One common approach involves the reaction of uracil with phenyl isocyanate under basic conditions. Another method includes the alkylation of 3-phenyluracils with alkylating agents while maintaining the pH between 1 and 6 .
Industrial Production Methods: Industrial production of this compound typically involves large-scale synthesis using optimized reaction conditions to ensure high yield and purity. The process may include steps such as purification through recrystallization or chromatography to obtain the desired product.
Chemical Reactions Analysis
Types of Reactions: 1-Phenyluracil undergoes various chemical reactions, including:
Oxidation: It can be oxidized to form corresponding oxo derivatives.
Reduction: Reduction reactions can yield dihydro derivatives.
Substitution: Substitution reactions, particularly at the 5 and 6 positions, can introduce various functional groups.
Common Reagents and Conditions:
Oxidation: Common oxidizing agents include potassium permanganate and hydrogen peroxide.
Reduction: Reducing agents such as sodium borohydride and lithium aluminum hydride are often used.
Substitution: Halogenation followed by nucleophilic substitution is a common method to introduce different substituents.
Major Products:
Oxidation: Produces oxo derivatives.
Reduction: Yields dihydro derivatives.
Substitution: Results in various substituted phenyluracil derivatives.
Scientific Research Applications
1-Phenyluracil has a wide range of applications in scientific research:
Chemistry: Used as a building block for the synthesis of more complex molecules.
Biology: Studied for its interactions with nucleic acids and proteins.
Medicine: Investigated for its potential therapeutic properties, including antiviral and anticancer activities.
Industry: Utilized in the development of herbicides and other agrochemicals
Comparison with Similar Compounds
Saflufenacil: Another phenyluracil derivative used as a herbicide.
Epyrifenacil: A novel phenyluracil herbicide with similar applications.
Uniqueness: 1-Phenyluracil is unique due to its specific structural features and the range of reactions it can undergo. Its versatility in synthetic chemistry and potential therapeutic applications set it apart from other similar compounds.
Properties
IUPAC Name |
1-phenylpyrimidine-2,4-dione | |
---|---|---|
Source | PubChem | |
URL | https://pubchem.ncbi.nlm.nih.gov | |
Description | Data deposited in or computed by PubChem | |
InChI |
InChI=1S/C10H8N2O2/c13-9-6-7-12(10(14)11-9)8-4-2-1-3-5-8/h1-7H,(H,11,13,14) | |
Source | PubChem | |
URL | https://pubchem.ncbi.nlm.nih.gov | |
Description | Data deposited in or computed by PubChem | |
InChI Key |
KELXKKNILLDRLS-UHFFFAOYSA-N | |
Source | PubChem | |
URL | https://pubchem.ncbi.nlm.nih.gov | |
Description | Data deposited in or computed by PubChem | |
Canonical SMILES |
C1=CC=C(C=C1)N2C=CC(=O)NC2=O | |
Source | PubChem | |
URL | https://pubchem.ncbi.nlm.nih.gov | |
Description | Data deposited in or computed by PubChem | |
Molecular Formula |
C10H8N2O2 | |
Source | PubChem | |
URL | https://pubchem.ncbi.nlm.nih.gov | |
Description | Data deposited in or computed by PubChem | |
DSSTOX Substance ID |
DTXSID10175596 | |
Record name | 1-Phenyluracil | |
Source | EPA DSSTox | |
URL | https://comptox.epa.gov/dashboard/DTXSID10175596 | |
Description | DSSTox provides a high quality public chemistry resource for supporting improved predictive toxicology. | |
Molecular Weight |
188.18 g/mol | |
Source | PubChem | |
URL | https://pubchem.ncbi.nlm.nih.gov | |
Description | Data deposited in or computed by PubChem | |
CAS No. |
21321-07-3 | |
Record name | 1-Phenyluracil | |
Source | ChemIDplus | |
URL | https://pubchem.ncbi.nlm.nih.gov/substance/?source=chemidplus&sourceid=0021321073 | |
Description | ChemIDplus is a free, web search system that provides access to the structure and nomenclature authority files used for the identification of chemical substances cited in National Library of Medicine (NLM) databases, including the TOXNET system. | |
Record name | 1-Phenyluracil | |
Source | EPA DSSTox | |
URL | https://comptox.epa.gov/dashboard/DTXSID10175596 | |
Description | DSSTox provides a high quality public chemistry resource for supporting improved predictive toxicology. | |
Synthesis routes and methods
Procedure details
Retrosynthesis Analysis
AI-Powered Synthesis Planning: Our tool employs the Template_relevance Pistachio, Template_relevance Bkms_metabolic, Template_relevance Pistachio_ringbreaker, Template_relevance Reaxys, Template_relevance Reaxys_biocatalysis model, leveraging a vast database of chemical reactions to predict feasible synthetic routes.
One-Step Synthesis Focus: Specifically designed for one-step synthesis, it provides concise and direct routes for your target compounds, streamlining the synthesis process.
Accurate Predictions: Utilizing the extensive PISTACHIO, BKMS_METABOLIC, PISTACHIO_RINGBREAKER, REAXYS, REAXYS_BIOCATALYSIS database, our tool offers high-accuracy predictions, reflecting the latest in chemical research and data.
Strategy Settings
Precursor scoring | Relevance Heuristic |
---|---|
Min. plausibility | 0.01 |
Model | Template_relevance |
Template Set | Pistachio/Bkms_metabolic/Pistachio_ringbreaker/Reaxys/Reaxys_biocatalysis |
Top-N result to add to graph | 6 |
Feasible Synthetic Routes
Q1: The studies mention novel ring transformations involving 1-phenyluracil derivatives. Could you elaborate on these reactions and their significance?
A1: Several studies showcase the unique reactivity of this compound derivatives:
- Reaction with amines and hydrazine: 5-Bromo-6-methyl-1-phenyluracil undergoes ring transformation to yield 1-arylhydantoins when treated with amines, and 4-ureidopyrazol-3-ones with hydrazine hydrate. This second transformation involves a fascinating double ring transformation via a hydantoin intermediate. [, ]
- Formation of barbituric acid derivatives: Both 5-thiocarbamoyl- and 5-carbamoyl-3-methyl-1-phenyluracil derivatives, when subjected to heat in ethanolic sodium ethoxide, undergo ring transformation to produce 5-anilinomethylene-4-thiobarbituric acid and 5-anilinomethylenebarbituric acids, respectively. []
Q2: Can you provide details about the synthesis of a specific this compound derivative mentioned in the papers?
A3: One paper details the synthesis of 6-(1-methylhydrazino)-1-phenyluracil, a key intermediate for synthesizing fervenulin-type compounds. [] This synthesis involves several steps:
- Reaction with methylhydrazine: Reacting compound (8) with methylhydrazine yields the desired 6-(1-methylhydrazino)-1-phenyluracil (16). []
Q3: How does the introduction of various substituents on the phenyl ring affect the biological activity of this compound?
A4: While specific structure-activity relationships (SAR) aren't extensively discussed in the provided papers, one study highlights that attaching appropriately substituted phenyl groups at the 1-position of uracil can dramatically increase its anticoccidial activity. [] This suggests that modifications to the phenyl ring can significantly impact the compound's potency, potentially by influencing its interaction with biological targets.
Disclaimer and Information on In-Vitro Research Products
Please be aware that all articles and product information presented on BenchChem are intended solely for informational purposes. The products available for purchase on BenchChem are specifically designed for in-vitro studies, which are conducted outside of living organisms. In-vitro studies, derived from the Latin term "in glass," involve experiments performed in controlled laboratory settings using cells or tissues. It is important to note that these products are not categorized as medicines or drugs, and they have not received approval from the FDA for the prevention, treatment, or cure of any medical condition, ailment, or disease. We must emphasize that any form of bodily introduction of these products into humans or animals is strictly prohibited by law. It is essential to adhere to these guidelines to ensure compliance with legal and ethical standards in research and experimentation.