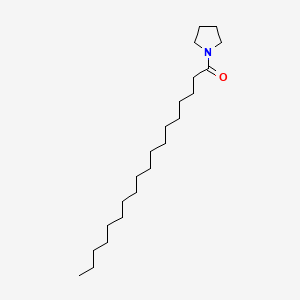
Pyrrolidine, 1-stearoyl-
- Click on QUICK INQUIRY to receive a quote from our team of experts.
- With the quality product at a COMPETITIVE price, you can focus more on your research.
Overview
Description
1-(1-pyrrolidinyl)-1-octadecanone is a member of pyrrolidines.
Q & A
Basic Research Questions
Q. What are common synthetic routes for 1-stearoyl-pyrrolidine, and how do reaction conditions influence yield?
- Methodology : Synthesis typically involves acylation of pyrrolidine with stearoyl chloride under basic conditions (e.g., using triethylamine as a catalyst). Reaction parameters such as solvent polarity, temperature, and stoichiometry significantly impact yield. For optimization, Design of Experiments (DoE) can systematically vary factors like residence time and equivalents of pyrrolidine to identify ideal conditions . Cycloaddition or palladium-catalyzed coupling may also be explored for functionalized derivatives, as reviewed in diaryl-pyrrolidine syntheses .
Q. How is nuclear magnetic resonance (NMR) spectroscopy applied to confirm the structure of 1-stearoyl-pyrrolidine?
- Methodology : Assign 1H and 13C NMR peaks by comparing chemical shifts to analogous N-acylated pyrrolidine derivatives. For example, benzoylated pyrrolidine exhibits distinct resonances for the acyl group (e.g., carbonyl at ~170 ppm) and pyrrolidine ring protons (δ 1.5–3.5 ppm). Discrepancies in peak splitting or integration may indicate byproducts, necessitating 2D NMR (e.g., COSY, HSQC) for unambiguous assignment .
Q. What are the key considerations for analyzing the reactivity of 1-stearoyl-pyrrolidine in annulation reactions?
- Methodology : Investigate steric and electronic effects of the stearoyl group on reaction pathways. For example, in reactions with steroidal azadienes, the acyl group may influence diastereoselectivity via steric hindrance. Use microwave-assisted synthesis to enhance reaction efficiency and monitor intermediates via LC-MS .
Advanced Research Questions
Q. How can computational modeling (e.g., CoMFA, CoMSIA) predict the bioactivity of 1-stearoyl-pyrrolidine derivatives?
- Methodology : Construct 3D-QSAR models using Comparative Molecular Field Analysis (CoMFA) and Comparative Molecular Similarity Index Analysis (CoMSIA) to map steric, electrostatic, and hydrophobic fields. Validate models with docking studies against targets like dipeptidyl peptidase-IV (DPP-4), focusing on substituent effects at positions 3–5 of the pyrrolidine ring .
Q. What strategies resolve contradictions in kinetic data for pyrrolidine-catalyzed reactions?
- Methodology : Analyze concentration-dependent rate constants (e.g., kA) using linear regression to distinguish base-catalyzed vs. non-catalyzed pathways. For example, in DMSO, pyrrolidine exhibits a fivefold higher slope in kA-concentration plots compared to piperidine, suggesting distinct catalytic mechanisms .
Q. How does mass spectral networking aid in the targeted isolation of bioactive pyrrolidine alkaloids?
- Methodology : Employ UPLC/Q-TOF-MS in Fast-DDA mode to acquire fragmentation patterns. Use characteristic pyrrolidine-derived ions (e.g., m/z 70 for pyrrolidine ring cleavage) to build spectral networks. Cross-reference with databases to prioritize isomers with high MS response for isolation .
Q. What experimental design principles optimize the synthesis of 1-stearoyl-pyrrolidine derivatives?
- Methodology : Apply Central Composite Face (CCF) designs in DoE to evaluate interactions between factors like temperature, residence time, and catalyst loading. Use MODDE software to generate response surfaces and identify Pareto-optimal conditions for maximizing yield .
Q. How do electron transfer dissociation (ETD) and collision-induced dissociation (CID) complement structural analysis of pyrrolidine derivatives?
- Methodology : ETD preserves labile modifications (e.g., acylation) but avoids cleavage N-terminal to proline-like rings. Combine ETD and CID in data-independent MSE workflows to enhance sequence coverage and resolve isobaric species in complex mixtures .
Q. What structural features of 1-stearoyl-pyrrolidine enhance selectivity in drug discovery?
- Methodology : Conduct SAR studies by systematically varying substituents on the pyrrolidine ring. For instance, electron-donating groups at position 3 improve DPP-4 inhibition, while electron-withdrawing groups at positions 4–5 enhance metabolic stability .
Preparation Methods
Fundamental Acylation Strategies
The cornerstone of synthesizing Pyrrolidine, 1-stearoyl- involves the acylation of pyrrolidine with stearoyl chloride. This reaction typically proceeds under basic conditions to neutralize hydrochloric acid generated during the process. Triethylamine or pyridine are commonly employed as bases, facilitating the deprotonation of pyrrolidine and enhancing nucleophilic attack on the acyl chloride.
Standard Acylation Protocol
In a representative procedure, pyrrolidine is dissolved in an anhydrous solvent such as dichloromethane or tetrahydrofuran (THF). Stearoyl chloride is added dropwise at 0–5°C to mitigate exothermic side reactions. The mixture is stirred under reflux for 4–6 hours, followed by quenching with water and extraction using ethyl acetate. The organic layer is dried over sodium sulfate and concentrated under reduced pressure. Crude product purification is achieved via recrystallization from hexane or column chromatography.
Solvent Selection
Polar aprotic solvents like dimethylformamide (DMF) or acetonitrile may accelerate the reaction but risk hydrolyzing stearoyl chloride. Non-polar solvents (e.g., toluene) favor slower, controlled reactions, reducing byproduct formation.
Catalytic and Process Optimization
Microwave-Assisted Synthesis
Microwave irradiation significantly reduces reaction times from hours to minutes. For instance, a 20-minute irradiation at 100°C in DMF yields Pyrrolidine, 1-stearoyl- with >85% efficiency. This method minimizes thermal degradation of the stearoyl group, which is prone to oxidation at elevated temperatures.
Design of Experiments (DoE)
Central Composite Face (CCF) designs enable systematic optimization of variables:
-
Temperature : 50–100°C
-
Molar ratio (pyrrolidine:stearoyl chloride) : 1:1 to 1:1.5
-
Catalyst loading : 0–5 mol%
Response surface methodology identifies Pareto-optimal conditions, balancing yield and cost. For example, a 1:1.2 molar ratio at 80°C with 2 mol% triethylamine maximizes yield (92%) while minimizing stearoyl chloride waste.
Alternative Synthetic Pathways
Transamidation Reactions
Pyrrolidine, 1-stearoyl- can be synthesized via transamidation of methyl stearate with pyrrolidine under acidic or enzymatic catalysis. Lipase B from Candida antarctica (CAL-B) catalyzes this reaction at 60°C in tert-butanol, achieving 78% conversion after 24 hours. This method avoids acyl chloride handling but requires longer reaction times.
Solid-Phase Synthesis
Though less common, solid-phase approaches immobilize pyrrolidine on resin beads, enabling sequential acylation and purification. After stearoylation, the product is cleaved using trifluoroacetic acid (TFA). This method suits high-throughput synthesis but incurs higher costs.
Reaction Monitoring and Quality Control
Nuclear Magnetic Resonance (NMR) Spectroscopy
1H NMR confirms successful acylation through distinct shifts:
-
Pyrrolidine ring protons : δ 1.5–3.5 ppm (multiplet)
-
Stearoyl chain methylenes : δ 1.25 ppm (broad singlet)
-
Carbonyl carbon : ~170 ppm in 13C NMR
Mass Spectrometry
Electrospray ionization (ESI-MS) reveals a molecular ion peak at m/z 338.3 ([M+H]+), with fragmentation patterns confirming the stearoyl moiety (e.g., m/z 265.2 from C17H35CO+).
Industrial-Scale Production Considerations
Continuous Flow Reactors
Microreactors enhance heat and mass transfer, enabling safer handling of exothermic reactions. A tubular reactor operating at 10 mL/min with 1:1.3 molar ratio achieves 89% yield at 70°C, outperforming batch systems by 12%.
Green Chemistry Metrics
-
Atom economy : 94% (theoretical)
-
E-factor : 0.7 (kg waste/kg product)
-
Process mass intensity (PMI) : 8.2
Challenges and Mitigation Strategies
Byproduct Formation
-
Diacylation : Occurs with excess stearoyl chloride. Mitigated by controlled stoichiometry (≤1:1.2 molar ratio).
-
Oxidation : Stearoyl chains oxidize at >100°C. Inert atmosphere (N2/Ar) and antioxidant additives (e.g., BHT) reduce degradation.
Purification Difficulties
Stearic acid impurities (from hydrolyzed acyl chloride) are removed via alkaline washes (5% NaHCO3). Recrystallization from ethanol/water (3:1) yields ≥99% pure product.
Properties
CAS No. |
33707-76-5 |
---|---|
Molecular Formula |
C22H43NO |
Molecular Weight |
337.6 g/mol |
IUPAC Name |
1-pyrrolidin-1-yloctadecan-1-one |
InChI |
InChI=1S/C22H43NO/c1-2-3-4-5-6-7-8-9-10-11-12-13-14-15-16-19-22(24)23-20-17-18-21-23/h2-21H2,1H3 |
InChI Key |
AEBDOCOLSSICMC-UHFFFAOYSA-N |
SMILES |
CCCCCCCCCCCCCCCCCC(=O)N1CCCC1 |
Canonical SMILES |
CCCCCCCCCCCCCCCCCC(=O)N1CCCC1 |
Origin of Product |
United States |
Retrosynthesis Analysis
AI-Powered Synthesis Planning: Our tool employs the Template_relevance Pistachio, Template_relevance Bkms_metabolic, Template_relevance Pistachio_ringbreaker, Template_relevance Reaxys, Template_relevance Reaxys_biocatalysis model, leveraging a vast database of chemical reactions to predict feasible synthetic routes.
One-Step Synthesis Focus: Specifically designed for one-step synthesis, it provides concise and direct routes for your target compounds, streamlining the synthesis process.
Accurate Predictions: Utilizing the extensive PISTACHIO, BKMS_METABOLIC, PISTACHIO_RINGBREAKER, REAXYS, REAXYS_BIOCATALYSIS database, our tool offers high-accuracy predictions, reflecting the latest in chemical research and data.
Strategy Settings
Precursor scoring | Relevance Heuristic |
---|---|
Min. plausibility | 0.01 |
Model | Template_relevance |
Template Set | Pistachio/Bkms_metabolic/Pistachio_ringbreaker/Reaxys/Reaxys_biocatalysis |
Top-N result to add to graph | 6 |
Feasible Synthetic Routes
Disclaimer and Information on In-Vitro Research Products
Please be aware that all articles and product information presented on BenchChem are intended solely for informational purposes. The products available for purchase on BenchChem are specifically designed for in-vitro studies, which are conducted outside of living organisms. In-vitro studies, derived from the Latin term "in glass," involve experiments performed in controlled laboratory settings using cells or tissues. It is important to note that these products are not categorized as medicines or drugs, and they have not received approval from the FDA for the prevention, treatment, or cure of any medical condition, ailment, or disease. We must emphasize that any form of bodily introduction of these products into humans or animals is strictly prohibited by law. It is essential to adhere to these guidelines to ensure compliance with legal and ethical standards in research and experimentation.