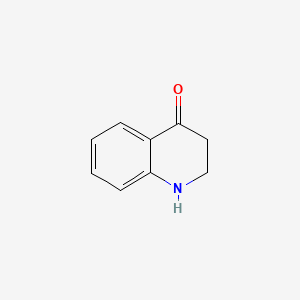
2,3-dihydro-1H-quinolin-4-one
Overview
Description
2,3-dihydro-1H-quinolin-4-one is a nitrogen-containing heterocyclic compound . It is a core structural component in various biologically active compounds . It is also used as a synthon for the preparation of biologically active quinazolinones and as a functional substrate for the synthesis of modified DHQ derivatives exhibiting different biological properties .
Synthesis Analysis
Several methodologies have been developed for the synthesis of the DHQ framework, especially the 2-substituted derivatives . A diversity-oriented synthesis of 2,2,3-substituted-2,3-dihydroquinolin-4 (1H)-ones vs. functionalised quinoline or N-alkenylindole derivatives through Brønsted acid mediated or Lewis acid catalyzed sequential reactions of 2-alkynylanilines with ketones has been described .Molecular Structure Analysis
The molecular formula of 2,3-dihydro-1H-quinolin-4-one is C9H9NO . The exact mass is 147.068413911 g/mol .Chemical Reactions Analysis
The cyclization reaction appears to proceed via regioselective rearrangement of the propargyl alcohol to an α,β-unsaturated ketone (Rupe rearrangement) and then 6-endo-trig ring closure (Donnelly-Farrell cyclization) .Physical And Chemical Properties Analysis
The physical and chemical properties of 2,3-dihydro-1H-quinolin-4-one include a molecular weight of 147.17 g/mol, XLogP3-AA of 1.4, Hydrogen Bond Donor Count of 1, Hydrogen Bond Acceptor Count of 2, Rotatable Bond Count of 0, Exact Mass of 147.068413911 g/mol, Monoisotopic Mass of 147.068413911 g/mol, Topological Polar Surface Area of 29.1 Ų, Heavy Atom Count of 11, and Formal Charge of 0 .Scientific Research Applications
Antileishmanial Agents
2,3-dihydro-1H-quinolin-4-one derivatives have been synthesized and evaluated for their antileishmanial efficacy against visceral leishmaniasis (VL). Among the library compounds, compound 5m exhibited potential in vitro antileishmanial activity .
Synthesis of Derivatives
This compound is used as a synthon for the preparation of biologically active quinazolinones and as a functional substrate for the synthesis of modified DHQ derivatives exhibiting different biological properties .
Antibacterial Activity
Antitumor Activity
Quinazolin-4(1H)-ones, which include 2,3-dihydro-1H-quinolin-4-one, have been found to have antitumor properties .
Antioxidant and Anticancer Activity
These compounds have also been studied for their antioxidant and anticancer activities .
Antifungal Activity
Research has shown that these compounds have potential antifungal properties .
Anticonvulsant Activity
Quinazolin-4(1H)-ones have been used in the development of anticonvulsant drugs .
Antihypertension Drugs
These compounds have been used in the development of antihypertension drugs .
Mechanism of Action
Target of Action
The primary target of 2,3-dihydro-1H-quinolin-4-one is the beta-adrenergic receptors . These receptors play a crucial role in the regulation of heart rate, smooth muscle tone, and metabolic rate.
Mode of Action
2,3-dihydro-1H-quinolin-4-one acts as a beta-adrenergic blocking agent . It binds to the beta-adrenergic receptors, blocking the binding of natural neurotransmitters like adrenaline. This prevents the activation of these receptors, thereby inhibiting the physiological responses to adrenaline.
Pharmacokinetics
One study has confirmed the stability of a similar compound in both simulated gastric fluid and simulated intestinal fluid , suggesting potential bioavailability.
Action Environment
The action, efficacy, and stability of 2,3-dihydro-1H-quinolin-4-one can be influenced by various environmental factors. For instance, the compound should be stored in a dry, well-ventilated place away from fire sources and oxidizing agents . The pH of the environment can also affect the compound’s stability and action .
Safety and Hazards
Future Directions
In recent years, the use of green chemistry and alternative strategies are being explored to prepare diverse DHQ derivatives . This compound is used as a synthon for the preparation of biologically active quinazolinones and as a functional substrate for the synthesis of modified DHQ derivatives exhibiting different biological properties .
properties
IUPAC Name |
2,3-dihydro-1H-quinolin-4-one | |
---|---|---|
Source | PubChem | |
URL | https://pubchem.ncbi.nlm.nih.gov | |
Description | Data deposited in or computed by PubChem | |
InChI |
InChI=1S/C9H9NO/c11-9-5-6-10-8-4-2-1-3-7(8)9/h1-4,10H,5-6H2 | |
Source | PubChem | |
URL | https://pubchem.ncbi.nlm.nih.gov | |
Description | Data deposited in or computed by PubChem | |
InChI Key |
BUWPZNOVIHAWHW-UHFFFAOYSA-N | |
Source | PubChem | |
URL | https://pubchem.ncbi.nlm.nih.gov | |
Description | Data deposited in or computed by PubChem | |
Canonical SMILES |
C1CNC2=CC=CC=C2C1=O | |
Source | PubChem | |
URL | https://pubchem.ncbi.nlm.nih.gov | |
Description | Data deposited in or computed by PubChem | |
Molecular Formula |
C9H9NO | |
Source | PubChem | |
URL | https://pubchem.ncbi.nlm.nih.gov | |
Description | Data deposited in or computed by PubChem | |
DSSTOX Substance ID |
DTXSID30276195 | |
Record name | 2,3-dihydro-1H-quinolin-4-one | |
Source | EPA DSSTox | |
URL | https://comptox.epa.gov/dashboard/DTXSID30276195 | |
Description | DSSTox provides a high quality public chemistry resource for supporting improved predictive toxicology. | |
Molecular Weight |
147.17 g/mol | |
Source | PubChem | |
URL | https://pubchem.ncbi.nlm.nih.gov | |
Description | Data deposited in or computed by PubChem | |
CAS RN |
4295-36-7 | |
Record name | 2,3-dihydro-1H-quinolin-4-one | |
Source | EPA DSSTox | |
URL | https://comptox.epa.gov/dashboard/DTXSID30276195 | |
Description | DSSTox provides a high quality public chemistry resource for supporting improved predictive toxicology. | |
Synthesis routes and methods I
Procedure details
Synthesis routes and methods II
Procedure details
Retrosynthesis Analysis
AI-Powered Synthesis Planning: Our tool employs the Template_relevance Pistachio, Template_relevance Bkms_metabolic, Template_relevance Pistachio_ringbreaker, Template_relevance Reaxys, Template_relevance Reaxys_biocatalysis model, leveraging a vast database of chemical reactions to predict feasible synthetic routes.
One-Step Synthesis Focus: Specifically designed for one-step synthesis, it provides concise and direct routes for your target compounds, streamlining the synthesis process.
Accurate Predictions: Utilizing the extensive PISTACHIO, BKMS_METABOLIC, PISTACHIO_RINGBREAKER, REAXYS, REAXYS_BIOCATALYSIS database, our tool offers high-accuracy predictions, reflecting the latest in chemical research and data.
Strategy Settings
Precursor scoring | Relevance Heuristic |
---|---|
Min. plausibility | 0.01 |
Model | Template_relevance |
Template Set | Pistachio/Bkms_metabolic/Pistachio_ringbreaker/Reaxys/Reaxys_biocatalysis |
Top-N result to add to graph | 6 |
Feasible Synthetic Routes
Q & A
Q1: What is the molecular formula and weight of 2,3-dihydroquinolin-4(1H)-one?
A1: The molecular formula of 2,3-dihydroquinolin-4(1H)-one is C9H9NO, and its molecular weight is 147.17 g/mol.
Q2: How can 2,3-dihydroquinolin-4(1H)-ones be synthesized?
A2: Several methods exist for synthesizing 2,3-dihydroquinolin-4(1H)-ones:
- Acid-catalyzed cyclization of 2-aminochalcones: This widely employed method involves the reaction of 2-aminoacetophenone with benzaldehyde derivatives under basic conditions to form 2-aminochalcones, followed by acid-catalyzed cyclization. [, , , , , , ]
- Palladium-catalyzed carbonylative cyclization: This method utilizes palladium catalysts and carbon monoxide to construct the dihydroquinolinone ring from N-(2-pyridyl)sulfonyl (N-SO2Py)-2-iodoanilines and terminal alkenes. [, ]
- Silver(I) triflate catalyzed synthesis: This mild and efficient one-pot procedure involves reacting o-aminoacetophenones and aromatic aldehydes in the presence of silver(I) triflate. []
- Tetrazole 5-acetic acid catalyzed synthesis: This method utilizes 1H-Tetrazole 5-acetic acid (TAA) as a catalyst for the synthesis of 2-aryl-2,3-dihydroquinolin-4(1H)-ones from 2’-aminoacetophenone under solvent-free conditions. []
- Other methods: Alternative synthetic approaches include the use of silica chloride, zirconyl nitrate, antimony trichloride, and indium(III) chloride as catalysts. [, , , , ]
Q3: What spectroscopic data are useful for characterizing 2,3-dihydroquinolin-4(1H)-ones?
A3: NMR spectroscopy (both 1H and 13C), IR spectroscopy, and mass spectrometry are commonly employed for characterizing these compounds. [, ] X-ray crystallography can also be used to determine the relative stereochemistry of 2,3-dihydroquinolin-4(1H)-one derivatives. []
Q4: What are some common chemical transformations of 2,3-dihydroquinolin-4(1H)-ones?
A4: 2,3-dihydroquinolin-4(1H)-ones can undergo various transformations, including:
- Halogenation: These compounds readily undergo regioselective and stereoselective α-halogenation at the 3-position using reagents like iodine or pyridinium perbromide. [, ]
- Suzuki-Miyaura cross-coupling: Palladium-catalyzed Suzuki-Miyaura reactions enable the introduction of aryl groups at the 6- and 8-positions of 2,3-dihydroquinolin-4(1H)-ones. []
- Oxidation: Treatment with thallium(III) p-tolylsulfonate or iodine can oxidize 2,3-dihydroquinolin-4(1H)-ones to the corresponding quinolin-4(1H)-ones or 4-methoxyquinolines, respectively. [, , ]
- Dehydrosulfonylation: 2-aryl-3-bromo-1-methanesulfonyl-2,3-dihydroquinolin-4(1H)-ones can undergo dimethyl sulfoxide (DMSO)-promoted dehydrosulfonylation to yield 2-aryl-3-bromoquinolin-4(1H)-ones. []
Q5: How do substituents on the 2,3-dihydroquinolin-4(1H)-one scaffold affect its reactivity?
A5: The nature and position of substituents can significantly influence the reactivity of the dihydroquinolinone core. For instance, electron-donating groups generally enhance reactivity towards electrophilic attack, while electron-withdrawing groups have the opposite effect. The presence of bulky substituents at the 2-position can hinder certain reactions due to steric hindrance. [, , ]
Q6: What are the known biological activities of 2,3-dihydroquinolin-4(1H)-ones?
A6: 2,3-Dihydroquinolin-4(1H)-ones exhibit a broad spectrum of biological activities, including:
- Anticancer activity: Several derivatives have demonstrated potent cytotoxic effects against various cancer cell lines, with some showing selectivity for cancer cells over normal cells. []
- Antifungal activity: Many 2,3-dihydroquinolin-4(1H)-one derivatives, particularly those containing semicarbazone moieties, display promising antifungal activity against various fungal strains. [, ]
- Antioxidant activity: Certain derivatives exhibit potent free radical scavenging ability, making them potential candidates for antioxidant therapies. []
- Antibacterial activity: Some 4-alkoxy-2-arylquinoline derivatives, synthesized from 2,3-dihydroquinolin-4(1H)-one precursors, show promising activity against Mycobacterium smegmatis, suggesting potential as antituberculosis agents. []
Q7: Are there any known drug candidates or pharmaceuticals based on the 2,3-dihydroquinolin-4(1H)-one scaffold?
A7: While no currently marketed drugs are solely based on the 2,3-dihydroquinolin-4(1H)-one core, this scaffold serves as a crucial building block in several drug discovery programs, particularly in anticancer and antimicrobial research. [, ]
Q8: What are the limitations of using 2,3-dihydroquinolin-4(1H)-ones as therapeutic agents?
A8: Despite their promising biological activities, several challenges need to be addressed before 2,3-dihydroquinolin-4(1H)-ones can be developed into viable therapeutics:
Q9: What are some other potential applications of 2,3-dihydroquinolin-4(1H)-ones beyond medicinal chemistry?
A9: Apart from their therapeutic potential, 2,3-dihydroquinolin-4(1H)-ones hold promise in diverse fields:
- Fluorescent probes: Derivatives exhibiting solvatochromic fluorescence emission properties show potential as sensors for specific analytes, such as fluoride ions. []
- Catalysts: The inherent chirality of certain 2,3-dihydroquinolin-4(1H)-ones makes them attractive candidates for developing chiral catalysts for asymmetric synthesis. []
Disclaimer and Information on In-Vitro Research Products
Please be aware that all articles and product information presented on BenchChem are intended solely for informational purposes. The products available for purchase on BenchChem are specifically designed for in-vitro studies, which are conducted outside of living organisms. In-vitro studies, derived from the Latin term "in glass," involve experiments performed in controlled laboratory settings using cells or tissues. It is important to note that these products are not categorized as medicines or drugs, and they have not received approval from the FDA for the prevention, treatment, or cure of any medical condition, ailment, or disease. We must emphasize that any form of bodily introduction of these products into humans or animals is strictly prohibited by law. It is essential to adhere to these guidelines to ensure compliance with legal and ethical standards in research and experimentation.