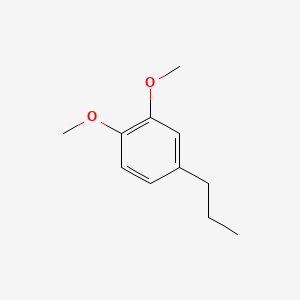
1,2-Dimethoxy-4-propylbenzene
Overview
Description
1,2-Dimethoxy-4-propylbenzene is an organic compound with the molecular formula C11H16O2. It is also known by other names such as dihydromethyleugenol and benzene, 1,2-dimethoxy-4-propyl . This compound is characterized by the presence of two methoxy groups (-OCH3) and a propyl group (-C3H7) attached to a benzene ring.
Mechanism of Action
Target of Action
The primary target of 1,2-Dimethoxy-4-propylbenzene is acetylcholinesterase , an enzyme that breaks down acetylcholine in the brain . Acetylcholine is a neurotransmitter involved in many functions, including muscle movement, breathing, heart rate, learning, and memory.
Mode of Action
This compound acts as a reversible inhibitor of acetylcholinesterase . It binds to the active site of the enzyme, preventing it from breaking down acetylcholine. This results in an increased concentration of acetylcholine in the brain, enhancing cholinergic neurotransmission .
Preparation Methods
Synthetic Routes and Reaction Conditions
1,2-Dimethoxy-4-propylbenzene can be synthesized through various methods. One common synthetic route involves the alkylation of 1,2-dimethoxybenzene with propyl bromide in the presence of a strong base such as potassium carbonate. The reaction typically takes place in an organic solvent like acetone or dimethylformamide at elevated temperatures .
Industrial Production Methods
In industrial settings, the production of this compound may involve continuous flow processes to ensure high yield and purity. Catalysts such as palladium on carbon (Pd/C) can be used to facilitate the reaction, and the process conditions are optimized to achieve efficient conversion rates .
Chemical Reactions Analysis
Types of Reactions
1,2-Dimethoxy-4-propylbenzene undergoes various chemical reactions, including:
Oxidation: The compound can be oxidized to form corresponding quinones or carboxylic acids using oxidizing agents like potassium permanganate or chromium trioxide.
Reduction: Reduction reactions can convert the compound to its corresponding alcohols or alkanes using reducing agents such as lithium aluminum hydride or hydrogen gas in the presence of a catalyst.
Substitution: Electrophilic aromatic substitution reactions can introduce different substituents onto the benzene ring.
Common Reagents and Conditions
Oxidation: Potassium permanganate (KMnO4), chromium trioxide (CrO3)
Reduction: Lithium aluminum hydride (LiAlH4), hydrogen gas (H2) with palladium on carbon (Pd/C)
Substitution: Bromine (Br2), chlorine (Cl2), nitric acid (HNO3)
Major Products Formed
Oxidation: Quinones, carboxylic acids
Reduction: Alcohols, alkanes
Substitution: Halogenated benzene derivatives, nitrobenzene derivatives
Scientific Research Applications
1,2-Dimethoxy-4-propylbenzene has several applications in scientific research:
Chemistry: It is used as a building block in organic synthesis to create more complex molecules.
Biology: The compound is studied for its potential biological activities, including antimicrobial and antioxidant properties.
Medicine: Research is ongoing to explore its potential therapeutic effects, such as anti-inflammatory and analgesic properties.
Industry: It is used in the production of fragrances and flavoring agents due to its pleasant aroma.
Comparison with Similar Compounds
Similar Compounds
1,2-Dimethoxy-4-propenylbenzene:
1,2-Dimethoxy-4-ethylbenzene: This compound has an ethyl group instead of a propyl group.
1,2-Dimethoxy-4-methylbenzene:
Uniqueness
1,2-Dimethoxy-4-propylbenzene is unique due to its specific substitution pattern on the benzene ring, which imparts distinct chemical and physical properties. Its combination of methoxy and propyl groups makes it a valuable intermediate in organic synthesis and a potential candidate for various biological and industrial applications .
Properties
IUPAC Name |
1,2-dimethoxy-4-propylbenzene | |
---|---|---|
Source | PubChem | |
URL | https://pubchem.ncbi.nlm.nih.gov | |
Description | Data deposited in or computed by PubChem | |
InChI |
InChI=1S/C11H16O2/c1-4-5-9-6-7-10(12-2)11(8-9)13-3/h6-8H,4-5H2,1-3H3 | |
Source | PubChem | |
URL | https://pubchem.ncbi.nlm.nih.gov | |
Description | Data deposited in or computed by PubChem | |
InChI Key |
YXLFQKUIZVSIEP-UHFFFAOYSA-N | |
Source | PubChem | |
URL | https://pubchem.ncbi.nlm.nih.gov | |
Description | Data deposited in or computed by PubChem | |
Canonical SMILES |
CCCC1=CC(=C(C=C1)OC)OC | |
Source | PubChem | |
URL | https://pubchem.ncbi.nlm.nih.gov | |
Description | Data deposited in or computed by PubChem | |
Molecular Formula |
C11H16O2 | |
Source | PubChem | |
URL | https://pubchem.ncbi.nlm.nih.gov | |
Description | Data deposited in or computed by PubChem | |
DSSTOX Substance ID |
DTXSID50207582 | |
Record name | 3,4-Dimethoxyphenylpropane | |
Source | EPA DSSTox | |
URL | https://comptox.epa.gov/dashboard/DTXSID50207582 | |
Description | DSSTox provides a high quality public chemistry resource for supporting improved predictive toxicology. | |
Molecular Weight |
180.24 g/mol | |
Source | PubChem | |
URL | https://pubchem.ncbi.nlm.nih.gov | |
Description | Data deposited in or computed by PubChem | |
CAS No. |
5888-52-8 | |
Record name | 1,2-Dimethoxy-4-propylbenzene | |
Source | CAS Common Chemistry | |
URL | https://commonchemistry.cas.org/detail?cas_rn=5888-52-8 | |
Description | CAS Common Chemistry is an open community resource for accessing chemical information. Nearly 500,000 chemical substances from CAS REGISTRY cover areas of community interest, including common and frequently regulated chemicals, and those relevant to high school and undergraduate chemistry classes. This chemical information, curated by our expert scientists, is provided in alignment with our mission as a division of the American Chemical Society. | |
Explanation | The data from CAS Common Chemistry is provided under a CC-BY-NC 4.0 license, unless otherwise stated. | |
Record name | 3,4-Dimethoxyphenylpropane | |
Source | ChemIDplus | |
URL | https://pubchem.ncbi.nlm.nih.gov/substance/?source=chemidplus&sourceid=0005888528 | |
Description | ChemIDplus is a free, web search system that provides access to the structure and nomenclature authority files used for the identification of chemical substances cited in National Library of Medicine (NLM) databases, including the TOXNET system. | |
Record name | 3,4-Dimethoxyphenylpropane | |
Source | EPA DSSTox | |
URL | https://comptox.epa.gov/dashboard/DTXSID50207582 | |
Description | DSSTox provides a high quality public chemistry resource for supporting improved predictive toxicology. | |
Record name | 4-PROPYLVERATROLE | |
Source | FDA Global Substance Registration System (GSRS) | |
URL | https://gsrs.ncats.nih.gov/ginas/app/beta/substances/V1O06LO267 | |
Description | The FDA Global Substance Registration System (GSRS) enables the efficient and accurate exchange of information on what substances are in regulated products. Instead of relying on names, which vary across regulatory domains, countries, and regions, the GSRS knowledge base makes it possible for substances to be defined by standardized, scientific descriptions. | |
Explanation | Unless otherwise noted, the contents of the FDA website (www.fda.gov), both text and graphics, are not copyrighted. They are in the public domain and may be republished, reprinted and otherwise used freely by anyone without the need to obtain permission from FDA. Credit to the U.S. Food and Drug Administration as the source is appreciated but not required. | |
Synthesis routes and methods
Procedure details
Retrosynthesis Analysis
AI-Powered Synthesis Planning: Our tool employs the Template_relevance Pistachio, Template_relevance Bkms_metabolic, Template_relevance Pistachio_ringbreaker, Template_relevance Reaxys, Template_relevance Reaxys_biocatalysis model, leveraging a vast database of chemical reactions to predict feasible synthetic routes.
One-Step Synthesis Focus: Specifically designed for one-step synthesis, it provides concise and direct routes for your target compounds, streamlining the synthesis process.
Accurate Predictions: Utilizing the extensive PISTACHIO, BKMS_METABOLIC, PISTACHIO_RINGBREAKER, REAXYS, REAXYS_BIOCATALYSIS database, our tool offers high-accuracy predictions, reflecting the latest in chemical research and data.
Strategy Settings
Precursor scoring | Relevance Heuristic |
---|---|
Min. plausibility | 0.01 |
Model | Template_relevance |
Template Set | Pistachio/Bkms_metabolic/Pistachio_ringbreaker/Reaxys/Reaxys_biocatalysis |
Top-N result to add to graph | 6 |
Feasible Synthetic Routes
Q1: What is the significance of 1,2-Dimethoxy-4-propylbenzene in the context of lignin degradation?
A1: this compound is identified as a product of lignin degradation in a study focusing on the catalytic cleavage of β-O-4 bonds in lignin model compounds []. The research demonstrates that using a metal-free solid acid zeolite catalyst in aqueous conditions under high hydrogen pressure can effectively break down veratrylglycero-β-guaiacyl ether (VGE), a lignin model compound. The formation of this compound, alongside other monomers like guaiacol, provides insights into the complex reaction pathways involved in lignin depolymerization. This finding is significant for developing efficient strategies to convert lignin into valuable chemicals and biofuels.
Q2: How does the structure of this compound influence its attractiveness to the Pacific fruit fly (Bactrocera xanthodes)?
A2: Research indicates that this compound, a novel eugenol analogue, exhibits a noticeable attractiveness to male Pacific fruit flies, although significantly less potent than methyl eugenol []. While the exact mechanism of attraction is not fully understood, this finding suggests that the structural features of this compound could play a role in the olfactory response of the flies. Further investigation into the structure-activity relationship could lead to the development of more effective and targeted attractants for monitoring and controlling this agricultural pest.
Q3: Can this compound be hydrogenated, and if so, what are the conditions and potential challenges?
A3: Yes, research shows that this compound can be hydrogenated using a water-soluble rhodium catalyst under mild conditions (25°C, 1 atm H2) in an aqueous ethanol medium []. The study highlights that the aromatic ring in this compound is less reactive towards hydrogenation compared to carbon-carbon double bonds or carbonyl groups present in other lignin model compounds. Additionally, the hydrogenation process can be accompanied by hydrogenolysis, leading to the removal of the methoxy (-OMe) group. Understanding these reactions and their selectivity is crucial for optimizing the conversion of lignin-derived compounds into desired products.
Disclaimer and Information on In-Vitro Research Products
Please be aware that all articles and product information presented on BenchChem are intended solely for informational purposes. The products available for purchase on BenchChem are specifically designed for in-vitro studies, which are conducted outside of living organisms. In-vitro studies, derived from the Latin term "in glass," involve experiments performed in controlled laboratory settings using cells or tissues. It is important to note that these products are not categorized as medicines or drugs, and they have not received approval from the FDA for the prevention, treatment, or cure of any medical condition, ailment, or disease. We must emphasize that any form of bodily introduction of these products into humans or animals is strictly prohibited by law. It is essential to adhere to these guidelines to ensure compliance with legal and ethical standards in research and experimentation.