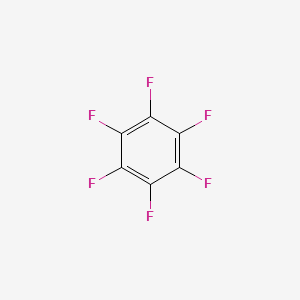
Hexafluorobenzene
Overview
Description
Hexafluorobenzene was repoted to be a sensitive 19F NMR indicator of tumor oxygenation. Rotational Raman spectra of hexafluorobenzenehas been studied under high resolution using a single mode argon laser as the exciting source. This compound in the gas phase reacts spontaneously with lithium amalgam, to give a solid and intimate mixture of lithium fluoride and elemental polymeric carbon with a small amount of superstoichiometric lithium. This compound forms series of 1:1 complexes with naphthalene, anthracene,phenanthrene, pyrene and triphenylene.
This compound is a member of the class of fluorobenzenes that is benzene in which all six hydrogen atom have been replaced by fluorine. It is a member of fluorobenzenes and a fluorocarbon.
Mechanism of Action
Target of Action
Hexafluorobenzene (HFB) is an organofluorine compound, a derivative of benzene where all hydrogen atoms have been replaced by fluorine atoms . It has been used as a reporter molecule to investigate tissue oxygenation in vivo . The primary targets of HFB are the tissues where oxygenation levels are being studied .
Mode of Action
HFB exhibits high gas solubility with ideal liquid-gas interactions . It interacts with its targets (tissues) by being exceedingly hydrophobic, allowing it to penetrate cellular membranes and reach hypoxic (low oxygen) regions . Since molecular oxygen is paramagnetic, it causes 19F NMR spin lattice relaxation (R1), specifically a linear dependence R1= a + bpO2 has been observed .
Biochemical Pathways
The photochemical isomerization and nonradiative decay processes of HFB have been investigated theoretically . On the S0 potential energy surface, HFB could isomerize to three different products . Following excitation to the S2 state with the perpendicular π → σ* transition, a chair-type minimum with Cs symmetry was found on the S2 potential energy surface . Both the S2 and S1 photochemistries of HFB yielded Dewar-HFB via the S1/S0 conical intersection .
Pharmacokinetics
HFB is a colorless liquid with a density of 1.6120 g/cm3 . It has a boiling point of 80.1 °C , suggesting that it can be readily absorbed and distributed in the body due to its liquid state at physiological temperatures. Its hydrophobic nature suggests that it may be metabolized and excreted slowly.
Result of Action
The molecular and cellular effects of HFB’s action primarily involve changes in tissue oxygenation .
Biochemical Analysis
Biochemical Properties
Hexafluorobenzene plays a role in biochemical reactions primarily due to its unique chemical structure. It is exceedingly hydrophobic and exhibits high gas solubility, making it useful as a reporter molecule in studies investigating tissue oxygenation in vivo . This compound interacts with various biomolecules, including enzymes and proteins, through nucleophilic aromatic substitution reactions. For example, it reacts with sodium hydrosulfide to produce pentafluorothiophenol . These interactions are crucial for understanding the compound’s behavior in biological systems.
Cellular Effects
This compound has been shown to cause various effects on different types of cells and cellular processes. It can cause eye and skin irritation, respiratory and digestive tract irritation, and central nervous system depression . Additionally, this compound has been identified as a neurotoxicant by the National Institute for Occupational Safety and Health (NIOSH) . These effects highlight the compound’s potential impact on cell signaling pathways, gene expression, and cellular metabolism.
Molecular Mechanism
The molecular mechanism of this compound involves its interactions at the molecular level, including binding interactions with biomolecules and enzyme inhibition or activation. This compound undergoes nucleophilic aromatic substitution reactions, which are essential for its biochemical activity . The compound’s ability to act as a molecular amplifier, due to its high solubility for oxygen, further influences its mechanism of action . These interactions can lead to changes in gene expression and cellular function.
Temporal Effects in Laboratory Settings
In laboratory settings, the effects of this compound can change over time. The compound is known for its stability and high gas solubility, which makes it suitable for long-term studies . Its hydrophobic nature and potential for causing tissue necrosis and blood clots in animal models indicate that its effects can vary depending on the duration of exposure . These temporal effects are important for understanding the compound’s long-term impact on cellular function.
Dosage Effects in Animal Models
The effects of this compound vary with different dosages in animal models. Studies have shown that high doses of this compound can lead to tissue necrosis, blood clots, and thrombosis . In contrast, lower doses may not exhibit these toxic effects. The compound’s impact on animal behavior, such as reduced mobility and muscle weakness, further underscores the importance of dosage in determining its safety and efficacy .
Metabolic Pathways
This compound is involved in various metabolic pathways, primarily through its interactions with enzymes and cofactors. The compound’s ability to undergo nucleophilic aromatic substitution reactions allows it to participate in metabolic processes . Additionally, this compound’s high gas solubility and hydrophobic nature influence its role in metabolic flux and metabolite levels . Understanding these pathways is crucial for elucidating the compound’s metabolic fate.
Transport and Distribution
This compound is transported and distributed within cells and tissues through interactions with transporters and binding proteins. Its hydrophobic nature and high gas solubility facilitate its movement across cell membranes . The compound’s localization and accumulation within specific tissues are influenced by these interactions, which are essential for its biological activity.
Subcellular Localization
The subcellular localization of this compound is determined by its chemical properties and interactions with cellular components. The compound’s hydrophobic nature allows it to accumulate in lipid-rich regions of the cell . Additionally, this compound’s interactions with specific targeting signals and post-translational modifications can direct it to particular compartments or organelles, influencing its activity and function.
Properties
IUPAC Name |
1,2,3,4,5,6-hexafluorobenzene | |
---|---|---|
Source | PubChem | |
URL | https://pubchem.ncbi.nlm.nih.gov | |
Description | Data deposited in or computed by PubChem | |
InChI |
InChI=1S/C6F6/c7-1-2(8)4(10)6(12)5(11)3(1)9 | |
Source | PubChem | |
URL | https://pubchem.ncbi.nlm.nih.gov | |
Description | Data deposited in or computed by PubChem | |
InChI Key |
ZQBFAOFFOQMSGJ-UHFFFAOYSA-N | |
Source | PubChem | |
URL | https://pubchem.ncbi.nlm.nih.gov | |
Description | Data deposited in or computed by PubChem | |
Canonical SMILES |
C1(=C(C(=C(C(=C1F)F)F)F)F)F | |
Source | PubChem | |
URL | https://pubchem.ncbi.nlm.nih.gov | |
Description | Data deposited in or computed by PubChem | |
Molecular Formula |
C6F6 | |
Source | PubChem | |
URL | https://pubchem.ncbi.nlm.nih.gov | |
Description | Data deposited in or computed by PubChem | |
Related CAS |
31668-87-8 | |
Record name | Benzene, 1,2,3,4,5,6-hexafluoro-, homopolymer | |
Source | CAS Common Chemistry | |
URL | https://commonchemistry.cas.org/detail?cas_rn=31668-87-8 | |
Description | CAS Common Chemistry is an open community resource for accessing chemical information. Nearly 500,000 chemical substances from CAS REGISTRY cover areas of community interest, including common and frequently regulated chemicals, and those relevant to high school and undergraduate chemistry classes. This chemical information, curated by our expert scientists, is provided in alignment with our mission as a division of the American Chemical Society. | |
Explanation | The data from CAS Common Chemistry is provided under a CC-BY-NC 4.0 license, unless otherwise stated. | |
DSSTOX Substance ID |
DTXSID5043924 | |
Record name | Hexafluorobenzene | |
Source | EPA DSSTox | |
URL | https://comptox.epa.gov/dashboard/DTXSID5043924 | |
Description | DSSTox provides a high quality public chemistry resource for supporting improved predictive toxicology. | |
Molecular Weight |
186.05 g/mol | |
Source | PubChem | |
URL | https://pubchem.ncbi.nlm.nih.gov | |
Description | Data deposited in or computed by PubChem | |
Physical Description |
Liquid; [Hawley] Colorless clear liquid; Melting point = 3.7-41 deg C; [MSDSonline] | |
Record name | Hexafluorobenzene | |
Source | Haz-Map, Information on Hazardous Chemicals and Occupational Diseases | |
URL | https://haz-map.com/Agents/8258 | |
Description | Haz-Map® is an occupational health database designed for health and safety professionals and for consumers seeking information about the adverse effects of workplace exposures to chemical and biological agents. | |
Explanation | Copyright (c) 2022 Haz-Map(R). All rights reserved. Unless otherwise indicated, all materials from Haz-Map are copyrighted by Haz-Map(R). No part of these materials, either text or image may be used for any purpose other than for personal use. Therefore, reproduction, modification, storage in a retrieval system or retransmission, in any form or by any means, electronic, mechanical or otherwise, for reasons other than personal use, is strictly prohibited without prior written permission. | |
Vapor Pressure |
84.5 [mmHg] | |
Record name | Hexafluorobenzene | |
Source | Haz-Map, Information on Hazardous Chemicals and Occupational Diseases | |
URL | https://haz-map.com/Agents/8258 | |
Description | Haz-Map® is an occupational health database designed for health and safety professionals and for consumers seeking information about the adverse effects of workplace exposures to chemical and biological agents. | |
Explanation | Copyright (c) 2022 Haz-Map(R). All rights reserved. Unless otherwise indicated, all materials from Haz-Map are copyrighted by Haz-Map(R). No part of these materials, either text or image may be used for any purpose other than for personal use. Therefore, reproduction, modification, storage in a retrieval system or retransmission, in any form or by any means, electronic, mechanical or otherwise, for reasons other than personal use, is strictly prohibited without prior written permission. | |
CAS No. |
392-56-3 | |
Record name | Hexafluorobenzene | |
Source | CAS Common Chemistry | |
URL | https://commonchemistry.cas.org/detail?cas_rn=392-56-3 | |
Description | CAS Common Chemistry is an open community resource for accessing chemical information. Nearly 500,000 chemical substances from CAS REGISTRY cover areas of community interest, including common and frequently regulated chemicals, and those relevant to high school and undergraduate chemistry classes. This chemical information, curated by our expert scientists, is provided in alignment with our mission as a division of the American Chemical Society. | |
Explanation | The data from CAS Common Chemistry is provided under a CC-BY-NC 4.0 license, unless otherwise stated. | |
Record name | Hexafluorobenzene | |
Source | ChemIDplus | |
URL | https://pubchem.ncbi.nlm.nih.gov/substance/?source=chemidplus&sourceid=0000392563 | |
Description | ChemIDplus is a free, web search system that provides access to the structure and nomenclature authority files used for the identification of chemical substances cited in National Library of Medicine (NLM) databases, including the TOXNET system. | |
Record name | HEXAFLUOROBENZENE | |
Source | DTP/NCI | |
URL | https://dtp.cancer.gov/dtpstandard/servlet/dwindex?searchtype=NSC&outputformat=html&searchlist=21628 | |
Description | The NCI Development Therapeutics Program (DTP) provides services and resources to the academic and private-sector research communities worldwide to facilitate the discovery and development of new cancer therapeutic agents. | |
Explanation | Unless otherwise indicated, all text within NCI products is free of copyright and may be reused without our permission. Credit the National Cancer Institute as the source. | |
Record name | Benzene, 1,2,3,4,5,6-hexafluoro- | |
Source | EPA Chemicals under the TSCA | |
URL | https://www.epa.gov/chemicals-under-tsca | |
Description | EPA Chemicals under the Toxic Substances Control Act (TSCA) collection contains information on chemicals and their regulations under TSCA, including non-confidential content from the TSCA Chemical Substance Inventory and Chemical Data Reporting. | |
Record name | Hexafluorobenzene | |
Source | EPA DSSTox | |
URL | https://comptox.epa.gov/dashboard/DTXSID5043924 | |
Description | DSSTox provides a high quality public chemistry resource for supporting improved predictive toxicology. | |
Record name | Hexafluorobenzene | |
Source | European Chemicals Agency (ECHA) | |
URL | https://echa.europa.eu/substance-information/-/substanceinfo/100.006.252 | |
Description | The European Chemicals Agency (ECHA) is an agency of the European Union which is the driving force among regulatory authorities in implementing the EU's groundbreaking chemicals legislation for the benefit of human health and the environment as well as for innovation and competitiveness. | |
Explanation | Use of the information, documents and data from the ECHA website is subject to the terms and conditions of this Legal Notice, and subject to other binding limitations provided for under applicable law, the information, documents and data made available on the ECHA website may be reproduced, distributed and/or used, totally or in part, for non-commercial purposes provided that ECHA is acknowledged as the source: "Source: European Chemicals Agency, http://echa.europa.eu/". Such acknowledgement must be included in each copy of the material. ECHA permits and encourages organisations and individuals to create links to the ECHA website under the following cumulative conditions: Links can only be made to webpages that provide a link to the Legal Notice page. | |
Record name | HEXAFLUOROBENZENE | |
Source | FDA Global Substance Registration System (GSRS) | |
URL | https://gsrs.ncats.nih.gov/ginas/app/beta/substances/CMC18T611K | |
Description | The FDA Global Substance Registration System (GSRS) enables the efficient and accurate exchange of information on what substances are in regulated products. Instead of relying on names, which vary across regulatory domains, countries, and regions, the GSRS knowledge base makes it possible for substances to be defined by standardized, scientific descriptions. | |
Explanation | Unless otherwise noted, the contents of the FDA website (www.fda.gov), both text and graphics, are not copyrighted. They are in the public domain and may be republished, reprinted and otherwise used freely by anyone without the need to obtain permission from FDA. Credit to the U.S. Food and Drug Administration as the source is appreciated but not required. | |
Retrosynthesis Analysis
AI-Powered Synthesis Planning: Our tool employs the Template_relevance Pistachio, Template_relevance Bkms_metabolic, Template_relevance Pistachio_ringbreaker, Template_relevance Reaxys, Template_relevance Reaxys_biocatalysis model, leveraging a vast database of chemical reactions to predict feasible synthetic routes.
One-Step Synthesis Focus: Specifically designed for one-step synthesis, it provides concise and direct routes for your target compounds, streamlining the synthesis process.
Accurate Predictions: Utilizing the extensive PISTACHIO, BKMS_METABOLIC, PISTACHIO_RINGBREAKER, REAXYS, REAXYS_BIOCATALYSIS database, our tool offers high-accuracy predictions, reflecting the latest in chemical research and data.
Strategy Settings
Precursor scoring | Relevance Heuristic |
---|---|
Min. plausibility | 0.01 |
Model | Template_relevance |
Template Set | Pistachio/Bkms_metabolic/Pistachio_ringbreaker/Reaxys/Reaxys_biocatalysis |
Top-N result to add to graph | 6 |
Feasible Synthetic Routes
Q1: What is the molecular formula and weight of hexafluorobenzene?
A1: this compound has a molecular formula of C6F6 and a molecular weight of 186.05 g/mol.
Q2: What spectroscopic data is available for this compound?
A2: Various spectroscopic techniques have been used to characterize this compound. These include:* NMR Spectroscopy: 1H and 19F NMR are particularly useful for studying this compound and its derivatives, including investigations of solute interactions [] and reaction monitoring [].* Rotational Spectroscopy: This method has been used to study the lone pair···π-hole interactions in complexes of this compound with tertiary alkylamines [].* Raman Spectroscopy: High-pressure Raman studies have provided insights into the phase transitions of this compound crystals []. * Photoelectron Spectroscopy: This technique has been applied to investigate hydrated this compound anions [].* UV-Vis Spectroscopy: UV-Vis spectrophotometry has been employed to study the association constants and enthalpies of formation for complexes formed by this compound with various amines [].
Q3: Does this compound exhibit any special optical properties?
A3: Yes, this compound exhibits a unique relationship between its static and optical-frequency polarizability anisotropies. Due to oppositely signed vibrational contributions, the ratio of static to optical-frequency anisotropy is greater than unity for this compound, unlike benzene, where it's less than unity [].
Q4: What is known about the structure of liquid this compound?
A4: Neutron and X-ray diffraction studies suggest that liquid this compound molecules tend to orient themselves in an L-type pair geometry. This arrangement changes to a nearly parallel alignment when this compound forms a 1:1 complex with benzene in the liquid phase [].
Q5: How does this compound interact with aromatic hydrocarbons?
A5: this compound forms complexes with aromatic hydrocarbons like benzene, toluene, and xylene. These interactions are characterized by a strong tendency for parallel orientation of the aromatic rings, suggesting significant contributions from quadrupole interactions []. The stability of these complexes increases with the number of methyl groups on the hydrocarbon ring [].
Q6: Can this compound act as a guest molecule in inclusion complexes?
A6: Yes, this compound can act as a guest molecule in inclusion complexes with certain host molecules. For example, it forms 2:1 inclusion complexes with bicyclic aryl-substituted sulfur-nitrogen compounds, primarily driven by π-stacking interactions of the arene-polyfluoroarene type [].
Q7: How does this compound interact with water?
A7: Ab initio studies suggest that this compound forms a weak dimer with water, with the oxygen atom of water interacting with the center of the aromatic ring [, ]. This interaction is different from the π-hydrogen bonding observed in the benzene-water dimer and is thought to arise from the electron-withdrawing effect of the fluorine atoms in this compound. Molecular dynamics simulations indicate that the equatorial region of this compound behaves like a typical hydrophobic solute, while the axial region exhibits unique solvation properties due to dominant pi-lone pair interactions with water [].
Q8: Does this compound form complexes with amines?
A8: Yes, this compound forms strong complexes with various amines, as indicated by the large exothermic heats of mixing and positive excess heat capacities observed experimentally []. Spectroscopic and thermodynamic studies have confirmed the formation of these complexes and provided insights into their association constants and enthalpies of formation [].
Q9: What is the nature of interaction between this compound and metal atoms?
A9: Metal atoms like ruthenium and osmium can form complexes with this compound, leading to unusual coordination modes where the this compound ligand is only partially bound (η4-coordination) [].
Q10: What types of reactions does this compound undergo?
A10: this compound is known to participate in a variety of reactions, including:* Photoaddition: It undergoes stereospecific photoreactions with alkenes and alkynes, yielding a range of cycloadducts depending on the reaction conditions and the specific reactant used [, , ].* Nucleophilic Substitution: Fluorine atoms in this compound can be replaced by nucleophiles, although this typically requires high temperatures or harsh conditions [].* C-F Bond Activation: Transition metal complexes can activate C-F bonds in this compound, leading to the formation of new organometallic compounds [, ].
Q11: How does this compound react under irradiation?
A11: this compound exhibits distinct photochemical behavior. Under UV irradiation, it can undergo photoaddition reactions with alkenes and alkynes, leading to various cycloadducts. The stereochemistry of these additions is highly dependent on the structure of the reacting partner and the reaction conditions [, , ]. For instance, cycloalkenes show a preference for [2+2] cycloaddition, with the stereoselectivity shifting from predominantly anti to syn as the ring size of the cycloalkene increases [].
Q12: How does gamma irradiation affect this compound?
A12: Gamma irradiation of liquid this compound leads to the formation of perfluoroheptane and polymers. Interestingly, when irradiated in the presence of benzene, the resulting polymer resembles that from pure benzene, suggesting a potential role for cyclohexadiene and cyclohexene units in its structure [].
Q13: Can this compound be used in the synthesis of nanoparticles?
A13: Yes, femtosecond laser pulses can be used to convert this compound into fluorine-doped hydrophilic carbon nanoparticles []. The fluorine doping enhances the water dispersibility of the nanoparticles, making them potentially useful for various applications.
Q14: What are the applications of this compound in peptide chemistry?
A14: this compound is a useful reagent for peptide stapling and cyclization through 1,4-selective thiol-fluoride substitution reactions []. This method allows for the creation of disulfide bonds within peptides, which can enhance their stability and bioactivity.
Q15: How is computational chemistry used to study this compound?
A15: Computational chemistry plays a crucial role in understanding the properties and reactivity of this compound. Various methods have been employed, including:* Density Functional Theory (DFT): DFT calculations have been used to study the electric and magnetic properties of this compound, providing insights into its polarizability, magnetizability, and hyperpolarizabilities [].* Ab initio Calculations: These high-level calculations have been used to investigate the intermolecular interactions of this compound with other molecules like benzene, water, and amines [, , ].* Molecular Dynamics Simulations: These simulations have provided information about the dynamic behavior of this compound in solution, particularly its interactions with water and its role in influencing the structure and dynamics of liquid mixtures [, ].* Monte Carlo Simulations: These simulations have been used to study the phase behavior of this compound mixtures, particularly the solid–fluid and solid–solid equilibrium in binary mixtures with benzene [].
Q16: What is known about the atmospheric degradation of this compound?
A16: this compound is primarily removed from the atmosphere through oxidation by hydroxyl radicals []. Theoretical studies have shown that this reaction proceeds through a prereaction complex and have provided insights into the reaction mechanism and kinetics [].
Q17: Is this compound considered a persistent organic pollutant?
A17: Yes, this compound has been identified as a potential persistent organic pollutant (POP) due to its relatively long tropospheric lifetime [].
Disclaimer and Information on In-Vitro Research Products
Please be aware that all articles and product information presented on BenchChem are intended solely for informational purposes. The products available for purchase on BenchChem are specifically designed for in-vitro studies, which are conducted outside of living organisms. In-vitro studies, derived from the Latin term "in glass," involve experiments performed in controlled laboratory settings using cells or tissues. It is important to note that these products are not categorized as medicines or drugs, and they have not received approval from the FDA for the prevention, treatment, or cure of any medical condition, ailment, or disease. We must emphasize that any form of bodily introduction of these products into humans or animals is strictly prohibited by law. It is essential to adhere to these guidelines to ensure compliance with legal and ethical standards in research and experimentation.