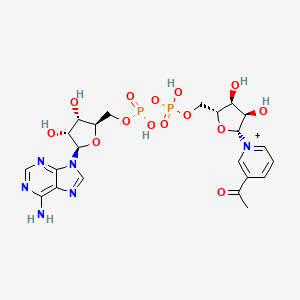
Apadh
- Click on QUICK INQUIRY to receive a quote from our team of experts.
- With the quality product at a COMPETITIVE price, you can focus more on your research.
Description
ApADH (3-Acetylpyridine Adenine Dinucleotide, reduced form) is a synthetic coenzyme analog of NADH (Nicotinamide Adenine Dinucleotide, reduced form), widely used in biochemical and enzymatic studies. Structurally, this compound replaces the nicotinamide moiety of NADH with a 3-acetylpyridine group, altering its redox properties and spectral characteristics . This modification enables this compound to participate in dehydrogenase-catalyzed reactions while offering distinct advantages in experimental settings, such as reduced spectral interference and simplified kinetic analysis .
This compound is particularly valuable in spectrophotometric assays where NADH or NADPH absorption at 340 nm overlaps with other components. This compound absorbs light at 363–375 nm (ε = 9.1 mM⁻¹ cm⁻¹), allowing for unambiguous detection in multi-enzyme systems . It is commercially available as a lyophilized powder with ≥92% purity and requires storage below 5°C to maintain stability .
Q & A
Basic Research Questions
Q. What is the biochemical role of APADH in enzymatic assays, and how does it differ from NADH?
this compound serves as an analog of NADH in redox reactions, with its oxidized form (APAD+) acting as a substrate for enzymes like transhydrogenase-2 (TH-2) and trypanothione reductase (TmTR). Unlike NADH, this compound exhibits a distinct redox potential (E<sup>07</sup> = –0.258 V) and absorbance peak at 375 nm, enabling specific detection in spectrophotometric assays . Its use avoids spectral overlap with NADH (340 nm), critical for multiplexed kinetic studies .
Q. What is the standard protocol for quantifying this compound in enzymatic activity assays?
this compound concentration is measured spectrophotometrically using its extinction coefficient (ε375 nm = 9.1 mM<sup>−1</sup> cm<sup>−1</sup>). For TH-2 activity, the reaction mixture (containing APAD+, NADH, and enzyme) is incubated at 37°C, and the rate of this compound formation is calculated as:
where W = sample weight, T = reaction time, and d = light path length .
Q. How does this compound’s redox potential influence its utility in thermodynamic studies?
this compound’s lower redox potential compared to NADH (E<sup>07</sup> = –0.320 V) makes it suitable for probing energetically unfavorable reactions. For instance, in TmTR-catalyzed reactions, this compound’s E<sup>07</sup> allows precise measurement of flavin adenine dinucleotide (FAD) redox states, revealing thermodynamically disfavored hydride transfers to disulfide bonds .
Advanced Research Questions
Q. How can researchers resolve contradictions in substrate inhibition patterns observed with APAD+/APADH systems?
In TmTR-catalyzed reactions, NADH exhibits substrate inhibition when APAD+ is present. This is resolved via Cleland plot linearization to determine inhibition constants (Ki). Competitive inhibition is confirmed if Ki increases with APAD+ concentration, indicating NADH binds to the reduced enzyme form. Pre-steady-state kinetics further distinguish binding order and rate-limiting steps .
Q. What methodological considerations are critical when designing pre-steady-state experiments with this compound?
Key considerations include:
- Rapid mixing : Use stopped-flow systems to capture transient intermediates (e.g., TmTR reduction by this compound occurs at kcat=1.3±0.3s−1).
- Wavelength selection : Monitor this compound at 363 nm (ε = 9.1 mM<sup>−1</sup> cm<sup>−1</sup>) to avoid interference from NADH or FAD.
- Data normalization : Correct for inner-filter effects in high-concentration assays .
Q. How do this compound-based assays address limitations in studying slow enzymatic substrates like glutaredoxin-1 (Grx-1)?
Grx-1’s slow reduction by TmTR (kcat=0.2–0.3s−1) complicates steady-state analysis. This compound’s distinct kinetics enable isolation of individual reaction phases. For example, this compound oxidation by TmTR (kcat/Km=3.2×105M−1s−1) provides a cleaner signal for quantifying disulfide bond reduction mechanisms .
Q. What statistical frameworks are recommended for analyzing this compound kinetic data with substrate inhibition?
Use non-linear regression to fit data to the uncompetitive substrate inhibition model:
Validate models via residual analysis and Akaike information criterion (AIC). For multi-substrate systems (e.g., NADH/APAD+), global fitting across substrate concentrations improves parameter accuracy .
Q. Methodological Best Practices
Q. How should researchers validate this compound purity and stability in long-term assays?
- Purity assessment : Use HPLC with UV detection (λ = 375 nm) and compare to reference spectra.
- Stability protocols : Store lyophilized this compound at ≤5°C, reconstitute in anaerobic buffers, and verify activity via control reactions before main experiments .
Q. What controls are essential when using this compound in coupled enzyme systems?
Include:
- Blank reactions : Omit substrate or enzyme to detect non-specific absorbance changes.
- Internal standards : Add known this compound concentrations to calibrate signal drift.
- Competitor assays : Use NADH to confirm this compound-specific activity .
Q. Data Interpretation and Reporting
Q. How can researchers reconcile discrepancies between this compound’s experimental and theoretical redox potentials?
Apply the Nernst equation using equilibrium constants (K) derived from forward/reverse kcat/Km ratios. For TmTR, K=8.68±1.54 yields E<sup>07</sup> = –0.230 V for FAD, aligning with independent calorimetric measurements .
Q. What criteria should guide the selection of this compound vs. NADH in mechanistic studies?
Choose this compound when:
- Spectral resolution is critical.
- Studying low-potential redox pairs.
- Avoiding NADH-linked side reactions (e.g., cytochrome interference).
Prioritize NADH for physiologically relevant models or high-turnover systems .
Comparison with Similar Compounds
Comparison of ApADH with Similar Compounds
Structural and Functional Comparison
Key Analogs:
NADH (Nicotinamide Adenine Dinucleotide, Reduced Form)
- Structure : Contains a nicotinamide ring.
- Absorption : λₘₐₓ = 340 nm (ε = 6.22 mM⁻¹ cm⁻¹).
- Function : Primary coenzyme in redox reactions, e.g., glycolysis and oxidative phosphorylation.
NADPH (Nicotinamide Adenine Dinucleotide Phosphate, Reduced Form)
- Structure : Similar to NADH but with an additional phosphate group.
- Absorption : λₘₐₓ = 340 nm (ε = 6.22 mM⁻¹ cm⁻¹).
- Function : Key reductant in anabolic pathways (e.g., lipid synthesis).
APAD⁺ (3-Acetylpyridine Adenine Dinucleotide, Oxidized Form)
- Structure : Oxidized form of this compound.
- Function : Substrate for dehydrogenases in assays measuring reductive activity .
Table 1: Comparative Properties of this compound and NADH
Mechanistic and Kinetic Differences
Hydride Transfer and Rate-Limiting Steps
This compound exhibits slower hydride transfer rates compared to NADH, making the transfer step more rate-limiting in reactions. For example:
- In mitochondrial complex I, the hydride transfer rate for this compound is >10× slower than NADH, with higher KIEs (~6.5 vs. ~3.5), suggesting greater transition-state stabilization .
- In lactate dehydrogenase (LDH) assays, this compound’s release from enzyme complexes is faster than NADH, simplifying kinetic analysis by reducing post-catalytic binding interference .
Substrate Inhibition and Binding Affinity
- This compound shows minimal substrate inhibition at high concentrations compared to NADH. This is attributed to weaker binding affinity to flavoproteins, which reduces competitive inhibition in multi-step reactions .
- Crystal structures of Plasmodium lactate dehydrogenase reveal that this compound and NADH bind similarly to the cofactor pocket, but this compound’s altered structure avoids steric clashes in human enzymes, enabling species-specific studies .
Advantages Over NADH/NADPH:
Spectral Resolution : this compound’s absorption at 375 nm avoids overlap with NAD(P)H at 340 nm, enabling simultaneous monitoring of multiple reactions .
Enzyme-Specific Activity Measurement :
- TH-2 (Transhydrogenase-2) Assays : ApAD⁺ is reduced to this compound, with activity quantified via 375 nm absorbance, bypassing NADH/NADPH interference .
- LDH (Lactate Dehydrogenase) Studies : this compound’s faster dissociation simplifies analysis of conformational changes during catalysis .
Limitations:
- Lower Catalytic Efficiency: Slower hydride transfer limits this compound’s use in high-throughput systems requiring rapid turnover .
- Cost and Stability : this compound is more expensive than NADH and degrades faster if improperly stored .
Research Findings and Case Studies
Mitochondrial Transhydrogenase Studies
This compound’s slower reaction kinetics in mitochondrial transhydrogenase (TH-2) revealed that hydride transfer and NAD⁺ dissociation are partially rate-limiting. This contrasts with NADH, where both steps are balanced, flattening the free energy profile .
Plasmodium Enzyme Inhibition
Structural studies of Plasmodium LDH-ApADH complexes identified conserved cofactor-binding pockets, enabling the design of inhibitors targeting both this compound and NADH binding sites .
Properties
Molecular Formula |
C22H29N6O14P2+ |
---|---|
Molecular Weight |
663.4 g/mol |
IUPAC Name |
[[(2R,3S,4R,5R)-5-(3-acetylpyridin-1-ium-1-yl)-3,4-dihydroxyoxolan-2-yl]methoxy-hydroxyphosphoryl] [(2R,3S,4R,5R)-5-(6-aminopurin-9-yl)-3,4-dihydroxyoxolan-2-yl]methyl hydrogen phosphate |
InChI |
InChI=1S/C22H28N6O14P2/c1-10(29)11-3-2-4-27(5-11)21-17(32)15(30)12(40-21)6-38-43(34,35)42-44(36,37)39-7-13-16(31)18(33)22(41-13)28-9-26-14-19(23)24-8-25-20(14)28/h2-5,8-9,12-13,15-18,21-22,30-33H,6-7H2,1H3,(H3-,23,24,25,34,35,36,37)/p+1/t12-,13-,15-,16-,17-,18-,21-,22-/m1/s1 |
InChI Key |
KPVQNXLUPNWQHM-RBEMOOQDSA-O |
Isomeric SMILES |
CC(=O)C1=C[N+](=CC=C1)[C@H]2[C@@H]([C@@H]([C@H](O2)COP(=O)(O)OP(=O)(O)OC[C@@H]3[C@H]([C@H]([C@@H](O3)N4C=NC5=C(N=CN=C54)N)O)O)O)O |
Canonical SMILES |
CC(=O)C1=C[N+](=CC=C1)C2C(C(C(O2)COP(=O)(O)OP(=O)(O)OCC3C(C(C(O3)N4C=NC5=C(N=CN=C54)N)O)O)O)O |
Synonyms |
3-acetyl-NAD 3-acetylpyridine adenine dinucleotide APAD APADH beta-3-acetylpyridine adenine dinucleotide |
Origin of Product |
United States |
Disclaimer and Information on In-Vitro Research Products
Please be aware that all articles and product information presented on BenchChem are intended solely for informational purposes. The products available for purchase on BenchChem are specifically designed for in-vitro studies, which are conducted outside of living organisms. In-vitro studies, derived from the Latin term "in glass," involve experiments performed in controlled laboratory settings using cells or tissues. It is important to note that these products are not categorized as medicines or drugs, and they have not received approval from the FDA for the prevention, treatment, or cure of any medical condition, ailment, or disease. We must emphasize that any form of bodily introduction of these products into humans or animals is strictly prohibited by law. It is essential to adhere to these guidelines to ensure compliance with legal and ethical standards in research and experimentation.