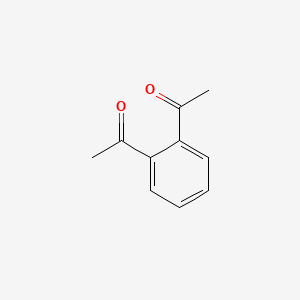
1,2-Diacetylbenzene
Overview
Description
1,2-Diacetylbenzene (1,2-DAB) is a neurotoxic aromatic γ-diketone and the primary metabolite of 1,2-diethylbenzene (1,2-DEB), a solvent found in gasoline and jet fuels . Upon exposure via dermal or inhalation routes, 1,2-DEB undergoes hepatic metabolism by cytochrome P-450 to yield 1,2-DAB, which accumulates in tissues and induces cytotoxicity, oxidative stress, and neurodegeneration . Key pathological effects include proximal neurofilament-filled axonopathy, limb weakness, and blue-green discoloration of tissues due to reactions with lysine residues in proteins .
Preparation Methods
Liquid-Phase Oxidation of 1,2-Diethylbenzene
The most industrially viable method for synthesizing 1,2-diacetylbenzene involves the liquid-phase oxidation of 1,2-diethylbenzene using molecular oxygen in the presence of water-soluble metal catalysts. This approach, detailed in GB1586843A , employs a biphasic reaction system to enhance selectivity and minimize by-product formation .
Reaction Mechanism and Conditions
The oxidation occurs in two sequential steps:
-
First Oxidation : Conversion of 1,2-diethylbenzene to 1-ethyl-2-acetylbenzene (ethylacetophenone).
-
Second Oxidation : Further oxidation of ethylacetophenone to this compound.
The process uses a heterogeneous mixture of:
-
Organic Phase : 25–98% by volume of 1,2-diethylbenzene or ethylacetophenone.
-
Aqueous Phase : 2–30% by volume containing 0.01–0.5 mol/L of water-soluble metal salts (e.g., cobalt(II) acetate, manganese acetate).
Key Parameters :
-
Temperature : 100–180°C
-
Pressure : 1–50 kg/cm²
-
pH : 1–6 (adjusted using sulfuric acid or ammonia)
Catalytic System and Selectivity
Cobalt(II) acetate demonstrates superior performance, achieving >95% recovery rates and ~79% selectivity toward diacetylbenzene . The aqueous phase facilitates catalyst separation, reducing decomposition during distillation.
Table 1: Performance of Catalysts in 1,2-Diethylbenzene Oxidation
Catalyst | Temperature (°C) | Selectivity (%) | Diacetylbenzene Yield (%) |
---|---|---|---|
Cobalt(II) acetate | 140 | 79 | 72 |
Manganese acetate | 130 | 68 | 61 |
Copper(II) sulfate | 150 | 55 | 49 |
Data adapted from Example 1 of GB1586843A .
While less common due to regioselectivity challenges, Friedel-Crafts acetylation can yield this compound under controlled conditions. This method requires strategic use of directing groups to overcome the inherent para preference of acetylating agents.
Directed Ortho Acetylation
Introducing a sterically hindering substituent (e.g., sulfonic acid group) at the meta position directs subsequent acetylation to the ortho position. For example:
-
Sulfonation : Treat o-xylene with fuming sulfuric acid to form o-xylene-4-sulfonic acid.
-
Acetylation : React with acetyl chloride/AlCl₃ to yield this compound-4-sulfonic acid.
-
Desulfonation : Hydrolyze under acidic conditions to remove the sulfonic acid group.
Limitations :
-
Low overall yield (~40%) due to multiple steps.
-
Requires harsh conditions that may degrade acetyl groups.
Oxidative Cleavage of 1,2-Divinylbenzene
A niche method involves the ozonolysis of 1,2-divinylbenzene followed by reductive workup:
-
Ozonolysis : React 1,2-divinylbenzene with ozone at −78°C to form an ozonide.
-
Reduction : Treat with dimethyl sulfide or zinc to produce this compound.
Advantages :
-
High regiochemical fidelity.
-
Suitable for small-scale laboratory synthesis.
Disadvantages :
-
Ozone handling requires specialized equipment.
-
Limited scalability for industrial use.
Comparative Analysis of Methods
Table 2: Method Comparison for this compound Synthesis
Method | Yield (%) | Selectivity (%) | Scalability | Cost Efficiency |
---|---|---|---|---|
Liquid-Phase Oxidation | 72 | 79 | High | Moderate |
Friedel-Crafts Acetylation | 40 | 65 | Low | Low |
Ozonolysis | 55 | 90 | Medium | High |
Optimization Strategies for Industrial Production
Catalyst Recycling
The aqueous phase in liquid-phase oxidation allows for 98% catalyst recovery via phase separation . Implementing continuous stirred-tank reactors (CSTRs) enhances throughput while maintaining selectivity.
Solvent Systems
Using polar aprotic solvents (e.g., dimethylformamide) in Friedel-Crafts reactions improves acetyl group stability, albeit at increased operational costs.
Process Intensification
Combining oxidation steps into a single reactor (one-step method) reduces equipment complexity but requires precise control over oxygen flow rates and temperature gradients .
Chemical Reactions Analysis
1,2-Diacetylbenzene undergoes various chemical reactions, including:
Oxidation: Involves aliphatic hydroxylation, epoxidation, oxidative dehydrogenation, and hydrogenation.
Reduction: Reduction reactions can convert this compound to its corresponding alcohols.
Common reagents used in these reactions include dimethyl sulfoxide, tetramethylammonium hydroxide, and various oxidizing agents. Major products formed from these reactions include cyclic enones and other oxidized derivatives .
Scientific Research Applications
Neurotoxic Research Applications
1,2-Diacetylbenzene has been extensively studied for its neurotoxic effects, particularly as a model compound to understand mechanisms of nerve damage and degeneration.
Case Study 1: Neurotoxicity Assessment
- Objective : To evaluate the neurotoxic effects of this compound on spinal cord tissues.
- Methodology : Adult male Sprague-Dawley rats were treated with varying doses of this compound.
- Findings : Significant alterations in protein expression related to axonal transport and cellular integrity were observed. The study concluded that this compound induces a dose-dependent increase in cellular damage markers .
Treatment Group | Dose (mg/kg) | Protein Expression Change | Observed Effects |
---|---|---|---|
Control | - | Baseline | No significant changes |
1,2-DAB | 20 | Increased | Axonal swelling and damage |
1,3-DAB | 20 | Minimal | No significant changes |
Case Study 2: Herbal Mitigation
- Objective : To assess the protective effects of silibinin against this compound toxicity.
- Methodology : Neuronal cultures were treated with both compounds.
- Findings : Silibinin treatment resulted in reduced ATP depletion and cell death compared to controls exposed solely to this compound .
Treatment | Cell Viability (%) | ATP Levels (µM) |
---|---|---|
Control | 100 | 5.0 |
1,2-DAB | 30 | 0.5 |
Silibinin + 1,2-DAB | 70 | 3.0 |
Industrial Applications
While primarily studied for its toxicological implications, this compound is also relevant in industrial settings where aromatic solvents are used. Its formation as a metabolite from solvents like 1,2-diethylbenzene raises concerns regarding occupational exposure and health risks associated with prolonged contact .
Potential Biomarker
Research suggests that the blue chromogen induced by exposure to this compound could serve as a urinary biomarker for diagnosing exposure to aromatic solvents . This aspect highlights its relevance not only in research but also in occupational health assessments.
Mechanism of Action
The mechanism of action of 1,2-diacetylbenzene involves its interaction with cytochrome P450 isoforms, particularly CYP1A2, leading to its metabolism and the formation of toxic metabolites . These metabolites can induce oxidative stress, apoptosis, and neuroendocrine disruption. The compound also affects mitochondrial function and axonal transport, leading to cellular ATP depletion and cell death .
Comparison with Similar Compounds
Structural Isomers: 1,2-DAB vs. 1,3-DAB
Mechanistic Insight : The ortho-diacetyl configuration in 1,2-DAB enables γ-diketone reactivity with lysine, forming pyrrole adducts that disrupt axonal transport . In contrast, the meta configuration of 1,3-DAB lacks this reactivity, rendering it inert .
Aliphatic vs. Aromatic γ-Diketones: 1,2-DAB vs. 2,5-Hexanedione (2,5-HD)
Mechanistic Insight : Both compounds share γ-diketone reactivity but differ in spatial targeting due to structural motifs. 1,2-DAB’s aromatic ring enhances tissue penetration and lysine-specific adduction, while 2,5-HD’s linear structure favors cysteine interactions in distal axons .
Other Benzene Derivatives: 1,2-DEB and 1,2,4-Triethylbenzene (1,2,4-TEB)
Key Finding : The 1,2-spaced ethyl/acetyl groups on benzene rings are critical for neurotoxicity, as seen in 1,2-DEB and 1,2-DAB. Modifying this arrangement (e.g., 1,3-DAB or 1,2,4-TEB) alters reactivity and toxicity .
Detailed Research Findings
Oxidative Stress and Cytotoxicity
- ROS Generation : 1,2-DAB induces apoptosis in HUVEC cells via ROS, reversible by antioxidants like N-acetylcysteine (NAC) .
- Neuronal Damage : In SH-SY5Y neuroblastoma cells, 1,2-DAB triggers oxidative stress, tau hyperphosphorylation, and impaired neurogenesis .
Molecular Targets
- αII-Spectrin Degradation : 1,2-DAB reduces αII-spectrin levels, destabilizing axonal integrity .
- Synaptic Proteins : Alters Syndig1l expression, impairing memory and motor function .
Therapeutic Insights
Biological Activity
1,2-Diacetylbenzene (DAB), also known as o-diacetylbenzene, is an aromatic compound that has garnered attention due to its significant biological activities and toxicological implications. This article provides a comprehensive overview of the biological activity of DAB, including its toxic effects, metabolic pathways, and potential mechanisms of action.
This compound is a gamma-diketone with the molecular formula C10H10O2. Its structure consists of a benzene ring with two acetyl groups attached at the ortho position. The compound's properties allow it to participate in various biochemical interactions, making it a subject of interest in toxicology and pharmacology.
Neurotoxicity
Research indicates that DAB exhibits neurotoxic effects. A study demonstrated that rats treated with DAB developed neurological symptoms such as limb weakness and blue discoloration of internal organs, particularly affecting the brain and spinal cord . The neurotoxic effects are attributed to the formation of neurofilament-filled axonal swellings, similar to those observed with other neurotoxic compounds .
Metabolic Pathways
An in silico study identified 13 metabolites of DAB resulting from both Phase I and Phase II metabolic reactions. These metabolites were shown to interact with cytochrome P450 isoforms, particularly CYP1A2, indicating their potential role in the metabolism of DAB . The metabolites exhibited harmful effects including:
- Neurotoxicity
- Nephrotoxicity
- Endocrine disruption
- Hepatotoxicity
These effects are linked to high gastrointestinal absorption and the ability to cross the blood-brain barrier .
DAB has been implicated in several biochemical pathways that contribute to its toxicity:
- Oxidative Stress : DAB and its metabolites can induce oxidative stress, leading to cellular damage and apoptosis.
- Caspase Activation : The compound activates caspases 3 and 8, which are crucial for apoptosis signaling pathways .
- Enzyme Inhibition : DAB acts as an inhibitor of superoxide dismutase and glutaminase, disrupting normal cellular functions .
Animal Studies
In a series of studies involving Sprague-Dawley rats, exposure to DAB resulted in significant neurotoxic effects characterized by behavioral changes and histopathological alterations in neural tissues . These findings highlight the compound's potential as a neurotoxin in both acute and chronic exposure scenarios.
Environmental Impact
DAB has also been studied for its environmental implications. It serves as a biomarker for exposure to aromatic solvents in marine organisms like the dab fish (Limanda limanda), which are used in monitoring programs for contaminants in aquatic environments . Elevated levels of ethoxyresorufin-O-deethylase (EROD) activity were observed in fish exposed to contaminated sediments, indicating sublethal chronic effects from compounds including DAB.
Data Summary
Biological Activity | Effect | Mechanism |
---|---|---|
Neurotoxicity | Limb weakness, blue discoloration | Neurofilament swelling |
Nephrotoxicity | Kidney damage | Oxidative stress |
Endocrine disruption | Hormonal imbalance | Metabolic interference |
Hepatotoxicity | Liver damage | Enzyme inhibition |
Q & A
Q. What are the established synthesis routes for 1,2-Diacetylbenzene, and how do their yields compare?
1,2-DAB can be synthesized via two primary methods using 1,2,4-trimethylnaphthalene as a precursor:
- Method 1 : Achieves a yield of ~50% under optimized conditions.
- Method 2 : Yields ~15%, likely due to competing side reactions or incomplete conversion.
Researchers should prioritize purification steps (e.g., column chromatography) to isolate 1,2-DAB from byproducts .
Q. How was 1,2-DAB identified as a neurotoxic metabolite, and what experimental models validated this?
1,2-DAB was identified as the neurotoxic metabolite of 1,2-diethylbenzene (DEB) through rodent studies. Oral administration of DEB (75–100 mg/kg) in Sprague-Dawley rats induced brainstem auditory evoked potential (BAEP) abnormalities, mirroring effects from direct 1,2-DAB exposure. Electrophysiological assessments and histopathological analysis of neural tissue confirmed axonal damage and neurofilament accumulation .
Q. Why does 1,2-DAB exhibit neurotoxicity while its structural isomer 1,3-DAB does not?
The gamma-diketone structure of 1,2-DAB enables covalent binding to lysine ε-amino groups in proteins, forming neurotoxic adducts (e.g., with neurofilaments). In contrast, 1,3-DAB (a delta-diketone) lacks this reactivity due to spatial constraints, preventing adduct formation and subsequent axonopathy .
Q. What methodologies are recommended for assessing age-dependent neurotoxic effects of 1,2-DAB?
- In vivo models : Intraperitoneal administration (3 mg/kg/day for 1 week) in young (6-month-old) and old (20-month-old) rats, followed by RNA-seq of hippocampal tissue.
- Key endpoints :
- Young rats : Upregulation of detoxification pathways (e.g., glutathione metabolism).
- Old rats : Activation of inflammatory pathways (e.g., TREM1 signaling linked to Alzheimer’s disease).
- Validation : RT-PCR for inflammatory markers (e.g., IL-6, TNF-α) and microglial activation assays .
Q. How does 1,2-DAB induce cytotoxicity in endothelial and neuronal cells?
1,2-DAB generates reactive oxygen species (ROS) in human umbilical vein endothelial cells (HUVECs), causing growth inhibition (4–8 μM) and apoptosis (16–32 μM). Pretreatment with antioxidants (e.g., N-acetylcysteine) mitigates cytotoxicity. In neuronal cultures, 1,2-DAB disrupts axonal transport by adduct formation with motor proteins (e.g., kinesin) and cytoskeletal components .
Q. What molecular pathways link 1,2-DAB exposure to Alzheimer’s disease (AD)-like pathology?
- Inflammatory pathways : TREM1 and NF-κB signaling are upregulated in aged rats, promoting neuroinflammation.
- Tau hyperphosphorylation : 1,2-DAB increases GSK-3β activity, leading to tau pathology and memory deficits.
- Oxidative stress : ROS generation accelerates age-related hippocampal neurodegeneration, mimicking AD progression .
Q. Can prolactin mitigate 1,2-DAB-induced neurotoxicity, and what mechanisms are involved?
Prolactin reduces motor and memory deficits in 1,2-DAB-treated rats by:
- Downregulating proinflammatory cytokines (IL-1β, IL-6).
- Modulating miRNA expression (e.g., miR-34a-5p) linked to synaptic plasticity.
- Enhancing hippocampal neurogenesis via STAT5 signaling pathways.
Experimental design: Co-administration of prolactin (0.5 mg/kg/day) with 1,2-DAB in young/old rats, followed by Morris water maze testing and miRNA profiling .
Q. What are the critical controls for in vitro studies of 1,2-DAB neurotoxicity?
- Structural controls : Include 1,3-DAB to isolate gamma-diketone-specific effects.
- Antioxidant controls : Use NAC or glutathione to confirm ROS-mediated toxicity.
- Cell-type specificity : Compare primary neurons, glia, and C6 glioma cells to identify cell-selective vulnerabilities .
Q. How does 1,2-DAB exposure alter miRNA profiles in the hippocampus?
Microarray analysis reveals:
- Upregulated miRNAs : miR-155-5p (proinflammatory) and miR-132-3p (synaptic plasticity).
- Downregulated miRNAs : miR-124-3p (neuroprotection) and miR-9-5p (neuronal differentiation).
Functional enrichment analysis links these miRNAs to axon guidance and MAPK signaling pathways .
Q. What are unresolved contradictions in 1,2-DAB research?
- Species sensitivity : Rodents show limb weakness, but human data are lacking despite occupational DEB exposure.
- Dose-response variability : Low-dose effects in aged models vs. high-dose acute toxicity in young animals.
- Therapeutic gaps : Prolactin’s efficacy in humans remains untested despite promising rodent data .
Properties
IUPAC Name |
1-(2-acetylphenyl)ethanone | |
---|---|---|
Source | PubChem | |
URL | https://pubchem.ncbi.nlm.nih.gov | |
Description | Data deposited in or computed by PubChem | |
InChI |
InChI=1S/C10H10O2/c1-7(11)9-5-3-4-6-10(9)8(2)12/h3-6H,1-2H3 | |
Source | PubChem | |
URL | https://pubchem.ncbi.nlm.nih.gov | |
Description | Data deposited in or computed by PubChem | |
InChI Key |
LVQFKRXRTXCQCZ-UHFFFAOYSA-N | |
Source | PubChem | |
URL | https://pubchem.ncbi.nlm.nih.gov | |
Description | Data deposited in or computed by PubChem | |
Canonical SMILES |
CC(=O)C1=CC=CC=C1C(=O)C | |
Source | PubChem | |
URL | https://pubchem.ncbi.nlm.nih.gov | |
Description | Data deposited in or computed by PubChem | |
Molecular Formula |
C10H10O2 | |
Source | PubChem | |
URL | https://pubchem.ncbi.nlm.nih.gov | |
Description | Data deposited in or computed by PubChem | |
DSSTOX Substance ID |
DTXSID20220667 | |
Record name | 1,2-Diacetylbenzene | |
Source | EPA DSSTox | |
URL | https://comptox.epa.gov/dashboard/DTXSID20220667 | |
Description | DSSTox provides a high quality public chemistry resource for supporting improved predictive toxicology. | |
Molecular Weight |
162.18 g/mol | |
Source | PubChem | |
URL | https://pubchem.ncbi.nlm.nih.gov | |
Description | Data deposited in or computed by PubChem | |
CAS No. |
704-00-7 | |
Record name | 1,2-Diacetylbenzene | |
Source | CAS Common Chemistry | |
URL | https://commonchemistry.cas.org/detail?cas_rn=704-00-7 | |
Description | CAS Common Chemistry is an open community resource for accessing chemical information. Nearly 500,000 chemical substances from CAS REGISTRY cover areas of community interest, including common and frequently regulated chemicals, and those relevant to high school and undergraduate chemistry classes. This chemical information, curated by our expert scientists, is provided in alignment with our mission as a division of the American Chemical Society. | |
Explanation | The data from CAS Common Chemistry is provided under a CC-BY-NC 4.0 license, unless otherwise stated. | |
Record name | 1,2-Diacetylbenzene | |
Source | ChemIDplus | |
URL | https://pubchem.ncbi.nlm.nih.gov/substance/?source=chemidplus&sourceid=0000704007 | |
Description | ChemIDplus is a free, web search system that provides access to the structure and nomenclature authority files used for the identification of chemical substances cited in National Library of Medicine (NLM) databases, including the TOXNET system. | |
Record name | 1,2-Diacetylbenzene | |
Source | EPA DSSTox | |
URL | https://comptox.epa.gov/dashboard/DTXSID20220667 | |
Description | DSSTox provides a high quality public chemistry resource for supporting improved predictive toxicology. | |
Record name | Benzene-1,2-bis(acetyl) | |
Source | European Chemicals Agency (ECHA) | |
URL | https://echa.europa.eu/substance-information/-/substanceinfo/100.010.799 | |
Description | The European Chemicals Agency (ECHA) is an agency of the European Union which is the driving force among regulatory authorities in implementing the EU's groundbreaking chemicals legislation for the benefit of human health and the environment as well as for innovation and competitiveness. | |
Explanation | Use of the information, documents and data from the ECHA website is subject to the terms and conditions of this Legal Notice, and subject to other binding limitations provided for under applicable law, the information, documents and data made available on the ECHA website may be reproduced, distributed and/or used, totally or in part, for non-commercial purposes provided that ECHA is acknowledged as the source: "Source: European Chemicals Agency, http://echa.europa.eu/". Such acknowledgement must be included in each copy of the material. ECHA permits and encourages organisations and individuals to create links to the ECHA website under the following cumulative conditions: Links can only be made to webpages that provide a link to the Legal Notice page. | |
Retrosynthesis Analysis
AI-Powered Synthesis Planning: Our tool employs the Template_relevance Pistachio, Template_relevance Bkms_metabolic, Template_relevance Pistachio_ringbreaker, Template_relevance Reaxys, Template_relevance Reaxys_biocatalysis model, leveraging a vast database of chemical reactions to predict feasible synthetic routes.
One-Step Synthesis Focus: Specifically designed for one-step synthesis, it provides concise and direct routes for your target compounds, streamlining the synthesis process.
Accurate Predictions: Utilizing the extensive PISTACHIO, BKMS_METABOLIC, PISTACHIO_RINGBREAKER, REAXYS, REAXYS_BIOCATALYSIS database, our tool offers high-accuracy predictions, reflecting the latest in chemical research and data.
Strategy Settings
Precursor scoring | Relevance Heuristic |
---|---|
Min. plausibility | 0.01 |
Model | Template_relevance |
Template Set | Pistachio/Bkms_metabolic/Pistachio_ringbreaker/Reaxys/Reaxys_biocatalysis |
Top-N result to add to graph | 6 |
Feasible Synthetic Routes
Disclaimer and Information on In-Vitro Research Products
Please be aware that all articles and product information presented on BenchChem are intended solely for informational purposes. The products available for purchase on BenchChem are specifically designed for in-vitro studies, which are conducted outside of living organisms. In-vitro studies, derived from the Latin term "in glass," involve experiments performed in controlled laboratory settings using cells or tissues. It is important to note that these products are not categorized as medicines or drugs, and they have not received approval from the FDA for the prevention, treatment, or cure of any medical condition, ailment, or disease. We must emphasize that any form of bodily introduction of these products into humans or animals is strictly prohibited by law. It is essential to adhere to these guidelines to ensure compliance with legal and ethical standards in research and experimentation.