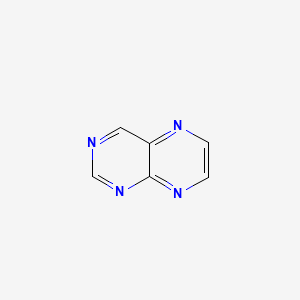
Pteridine
Overview
Description
Pteridine is a bicyclic heterocyclic compound consisting of fused pyrazine and pyrimidine rings. It is a fundamental structure in various biological pigments and enzyme cofactors. Pteridines are widely distributed in nature and play crucial roles in biological processes, including the biosynthesis of folic acid and other essential cofactors .
Mechanism of Action
Target of Action
Pteridine primarily targets the enzyme This compound Reductase 1 (PTR1) . PTR1 is a folate and pterin pathway enzyme unique for pathogenic trypanosomatids . As a validated drug target, PTR1 has been the focus of recent research efforts aimed at finding more effective treatments against human parasitic diseases such as leishmaniasis or sleeping sickness .
Mode of Action
This compound interacts with its target, PTR1, by binding to the active site of the enzyme . This interaction affects the enzyme’s dynamics, impacting the binding of natural substrates and inhibitors . The flexible regions around the active site, highly conserved structural waters, and species-specific differences in pocket properties and dynamics are key characteristics of PTR1 that likely impact the binding of this compound .
Biochemical Pathways
Pteridines are metabolic intermediates in the biosynthetic pathways of various cofactors and vitamins . They play essential roles in diverse biological activities ranging from immune system modulation, cellular signaling, coloration, and metabolism . This compound metabolism becomes disrupted in certain conditions, such as breast cancer . Several pteridines, including pterin, isoxanthopterin, xanthopterin, sepiapterin, 6-biopterin, lumazine, and 7-hydroxylumazine, have altered metabolism in breast cancer cell lines .
Pharmacokinetics
Research has shown that several pteridines are metabolized in breast cancer cell lines . More research is needed to fully understand the ADME properties of this compound and their impact on its bioavailability.
Result of Action
The result of this compound’s action is primarily seen in its influence on the function of the PTR1 enzyme and the subsequent effects on the folate and pterin pathways . The disruption of this compound metabolism in certain diseases, such as breast cancer, highlights the molecular and cellular effects of this compound’s action .
Action Environment
The action of this compound can be influenced by various environmental factors. For instance, in bacteria, pterins have been implicated in phenotypes related to UV protection and phototaxis .
Biochemical Analysis
Biochemical Properties
Pteridine is involved in several biochemical reactions, primarily as a cofactor. It interacts with enzymes such as sulfite oxidase, nitrate reductase, and dimethyl sulfoxide reductase, which are essential for bacterial metabolism and global nutrient cycles . This compound also plays a role in the metabolism of aromatic amino acids and cyanide utilization in bacteria . The nature of these interactions often involves the binding of this compound to the active sites of these enzymes, facilitating redox reactions and other catalytic processes.
Cellular Effects
This compound influences various cellular processes, including immune system modulation, cellular signaling, and metabolism . In cancer cells, altered this compound metabolism has been observed, with specific pteridines like pterin and sepiapterin showing differential expression related to tumorigenicity . This compound also affects cell signaling pathways and gene expression, contributing to changes in cellular metabolism and function.
Molecular Mechanism
At the molecular level, this compound exerts its effects through binding interactions with biomolecules. For instance, this compound reductase 1 (PTR1) is an enzyme that reduces pterins and folates, playing a crucial role in the survival of trypanosomatid parasites . This compound’s interactions with enzymes often involve nucleophilic attacks and redox reactions, which are essential for its function as a cofactor .
Temporal Effects in Laboratory Settings
In laboratory settings, the effects of this compound can change over time. Studies have shown that this compound levels can be altered in response to various pathophysiological processes . The stability and degradation of this compound, as well as its long-term effects on cellular function, are important considerations in both in vitro and in vivo studies .
Dosage Effects in Animal Models
The effects of this compound vary with different dosages in animal models. High doses of this compound can lead to toxic or adverse effects, while lower doses may be beneficial for certain metabolic processes . The threshold effects observed in these studies highlight the importance of dosage in determining the overall impact of this compound on animal health.
Metabolic Pathways
This compound is involved in several metabolic pathways, including the biosynthesis of folate and tetrahydrobiopterin . These pathways are crucial for the metabolism of amino acids and the production of neurotransmitters. This compound interacts with enzymes such as dihydropteroate synthase and dihydrofolate reductase, which are essential for its metabolic functions .
Transport and Distribution
Within cells and tissues, this compound is transported and distributed through various mechanisms. It interacts with transporters and binding proteins that facilitate its movement across cell membranes . The localization and accumulation of this compound within specific cellular compartments are influenced by these interactions.
Subcellular Localization
This compound’s subcellular localization is determined by targeting signals and post-translational modifications that direct it to specific compartments or organelles . These localization patterns can affect this compound’s activity and function, influencing its role in cellular processes.
Preparation Methods
Synthetic Routes and Reaction Conditions: Pteridine can be synthesized through several methods, primarily starting from pyrimidine or pyrazine derivatives. One common synthetic route involves the condensation of 4,5-diaminopyrimidine with glyoxal under acidic conditions to form the this compound ring system .
Industrial Production Methods: Industrial production of this compound often involves large-scale synthesis using optimized reaction conditions to ensure high yield and purity. The process typically includes steps such as purification through recrystallization and characterization using spectroscopic techniques .
Chemical Reactions Analysis
Types of Reactions: Pteridine undergoes various chemical reactions, including:
Oxidation: this compound can be oxidized to form pterin derivatives.
Reduction: Reduction of this compound leads to the formation of dihydrothis compound and tetrahydrothis compound.
Substitution: Nucleophilic substitution reactions can occur at the ring nitrogens and carbons.
Common Reagents and Conditions:
Oxidation: Common oxidizing agents include hydrogen peroxide and potassium permanganate.
Reduction: Reducing agents such as sodium borohydride and lithium aluminum hydride are used.
Substitution: Nucleophiles like amines and thiols are often employed in substitution reactions.
Major Products:
Oxidation: Pterin derivatives.
Reduction: Dihydrothis compound and tetrahydrothis compound.
Substitution: Various substituted pteridines depending on the nucleophile used.
Scientific Research Applications
Pteridine and its derivatives have numerous applications in scientific research:
Comparison with Similar Compounds
Pteridine is structurally similar to other heterocyclic compounds such as:
Pterin: A derivative of this compound with additional functional groups.
Flavin: Another bicyclic compound involved in redox reactions.
Pyrazine and Pyrimidine: The parent structures of this compound.
Uniqueness: this compound’s unique structure allows it to participate in a wide range of biological and chemical processes, making it a versatile compound in various fields of research and industry .
Properties
IUPAC Name |
pteridine | |
---|---|---|
Source | PubChem | |
URL | https://pubchem.ncbi.nlm.nih.gov | |
Description | Data deposited in or computed by PubChem | |
InChI |
InChI=1S/C6H4N4/c1-2-9-6-5(8-1)3-7-4-10-6/h1-4H | |
Source | PubChem | |
URL | https://pubchem.ncbi.nlm.nih.gov | |
Description | Data deposited in or computed by PubChem | |
InChI Key |
CPNGPNLZQNNVQM-UHFFFAOYSA-N | |
Source | PubChem | |
URL | https://pubchem.ncbi.nlm.nih.gov | |
Description | Data deposited in or computed by PubChem | |
Canonical SMILES |
C1=CN=C2C(=N1)C=NC=N2 | |
Source | PubChem | |
URL | https://pubchem.ncbi.nlm.nih.gov | |
Description | Data deposited in or computed by PubChem | |
Molecular Formula |
C6H4N4 | |
Source | PubChem | |
URL | https://pubchem.ncbi.nlm.nih.gov | |
Description | Data deposited in or computed by PubChem | |
DSSTOX Substance ID |
DTXSID60238347 | |
Record name | Pteridine | |
Source | EPA DSSTox | |
URL | https://comptox.epa.gov/dashboard/DTXSID60238347 | |
Description | DSSTox provides a high quality public chemistry resource for supporting improved predictive toxicology. | |
Molecular Weight |
132.12 g/mol | |
Source | PubChem | |
URL | https://pubchem.ncbi.nlm.nih.gov | |
Description | Data deposited in or computed by PubChem | |
CAS No. |
91-18-9 | |
Record name | Pteridine | |
Source | CAS Common Chemistry | |
URL | https://commonchemistry.cas.org/detail?cas_rn=91-18-9 | |
Description | CAS Common Chemistry is an open community resource for accessing chemical information. Nearly 500,000 chemical substances from CAS REGISTRY cover areas of community interest, including common and frequently regulated chemicals, and those relevant to high school and undergraduate chemistry classes. This chemical information, curated by our expert scientists, is provided in alignment with our mission as a division of the American Chemical Society. | |
Explanation | The data from CAS Common Chemistry is provided under a CC-BY-NC 4.0 license, unless otherwise stated. | |
Record name | Pteridine | |
Source | ChemIDplus | |
URL | https://pubchem.ncbi.nlm.nih.gov/substance/?source=chemidplus&sourceid=0000091189 | |
Description | ChemIDplus is a free, web search system that provides access to the structure and nomenclature authority files used for the identification of chemical substances cited in National Library of Medicine (NLM) databases, including the TOXNET system. | |
Record name | PTERIDINE | |
Source | DTP/NCI | |
URL | https://dtp.cancer.gov/dtpstandard/servlet/dwindex?searchtype=NSC&outputformat=html&searchlist=268562 | |
Description | The NCI Development Therapeutics Program (DTP) provides services and resources to the academic and private-sector research communities worldwide to facilitate the discovery and development of new cancer therapeutic agents. | |
Explanation | Unless otherwise indicated, all text within NCI products is free of copyright and may be reused without our permission. Credit the National Cancer Institute as the source. | |
Record name | Pteridine | |
Source | EPA DSSTox | |
URL | https://comptox.epa.gov/dashboard/DTXSID60238347 | |
Description | DSSTox provides a high quality public chemistry resource for supporting improved predictive toxicology. | |
Record name | PTERIDINE | |
Source | FDA Global Substance Registration System (GSRS) | |
URL | https://gsrs.ncats.nih.gov/ginas/app/beta/substances/6EZF26XQ81 | |
Description | The FDA Global Substance Registration System (GSRS) enables the efficient and accurate exchange of information on what substances are in regulated products. Instead of relying on names, which vary across regulatory domains, countries, and regions, the GSRS knowledge base makes it possible for substances to be defined by standardized, scientific descriptions. | |
Explanation | Unless otherwise noted, the contents of the FDA website (www.fda.gov), both text and graphics, are not copyrighted. They are in the public domain and may be republished, reprinted and otherwise used freely by anyone without the need to obtain permission from FDA. Credit to the U.S. Food and Drug Administration as the source is appreciated but not required. | |
Synthesis routes and methods
Procedure details
Retrosynthesis Analysis
AI-Powered Synthesis Planning: Our tool employs the Template_relevance Pistachio, Template_relevance Bkms_metabolic, Template_relevance Pistachio_ringbreaker, Template_relevance Reaxys, Template_relevance Reaxys_biocatalysis model, leveraging a vast database of chemical reactions to predict feasible synthetic routes.
One-Step Synthesis Focus: Specifically designed for one-step synthesis, it provides concise and direct routes for your target compounds, streamlining the synthesis process.
Accurate Predictions: Utilizing the extensive PISTACHIO, BKMS_METABOLIC, PISTACHIO_RINGBREAKER, REAXYS, REAXYS_BIOCATALYSIS database, our tool offers high-accuracy predictions, reflecting the latest in chemical research and data.
Strategy Settings
Precursor scoring | Relevance Heuristic |
---|---|
Min. plausibility | 0.01 |
Model | Template_relevance |
Template Set | Pistachio/Bkms_metabolic/Pistachio_ringbreaker/Reaxys/Reaxys_biocatalysis |
Top-N result to add to graph | 6 |
Feasible Synthetic Routes
Disclaimer and Information on In-Vitro Research Products
Please be aware that all articles and product information presented on BenchChem are intended solely for informational purposes. The products available for purchase on BenchChem are specifically designed for in-vitro studies, which are conducted outside of living organisms. In-vitro studies, derived from the Latin term "in glass," involve experiments performed in controlled laboratory settings using cells or tissues. It is important to note that these products are not categorized as medicines or drugs, and they have not received approval from the FDA for the prevention, treatment, or cure of any medical condition, ailment, or disease. We must emphasize that any form of bodily introduction of these products into humans or animals is strictly prohibited by law. It is essential to adhere to these guidelines to ensure compliance with legal and ethical standards in research and experimentation.