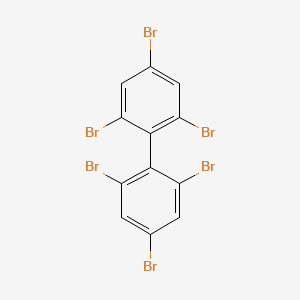
2,2',4,4',6,6'-Hexabromobiphenyl
Overview
Description
2,2’,4,4’,6,6’-Hexabromobiphenyl is a chemical compound belonging to the group of polybrominated biphenyls. It is characterized by its high molecular weight of 627.584 g/mol and its molecular formula C12H4Br6 . This compound is known for its persistence in the environment and its bioaccumulative properties, making it a significant environmental pollutant . Historically, it has been used as a flame retardant in various industrial applications .
Mechanism of Action
- Upon binding to AhR, HBB activates the expression of multiple phase I and II xenobiotic chemical metabolizing enzyme genes, including CYP1A1 .
- This binding initiates transcription, resulting in increased expression of enzymes involved in xenobiotic metabolism .
Target of Action
Mode of Action
Biochemical Pathways
Pharmacokinetics
Result of Action
Action Environment
Biochemical Analysis
Biochemical Properties
2,2’,4,4’,6,6’-Hexabromobiphenyl plays a significant role in biochemical reactions, particularly in the context of its interactions with enzymes and proteins. This compound is known to interact with cytochrome P450 enzymes, specifically CYP1A1, which are involved in the metabolism of xenobiotics . The interaction between 2,2’,4,4’,6,6’-Hexabromobiphenyl and CYP1A1 leads to the activation of phase I and II xenobiotic metabolizing enzymes, which are crucial for the detoxification and elimination of harmful substances from the body . Additionally, 2,2’,4,4’,6,6’-Hexabromobiphenyl can bind to the aryl hydrocarbon receptor (AhR), a ligand-activated transcription factor that regulates the expression of various genes involved in xenobiotic metabolism .
Cellular Effects
The effects of 2,2’,4,4’,6,6’-Hexabromobiphenyl on cellular processes are profound and multifaceted. This compound has been shown to influence cell signaling pathways, gene expression, and cellular metabolism. For instance, the binding of 2,2’,4,4’,6,6’-Hexabromobiphenyl to the AhR receptor leads to the activation of the AhR signaling pathway, which in turn modulates the expression of genes involved in cell cycle regulation and apoptosis . Furthermore, 2,2’,4,4’,6,6’-Hexabromobiphenyl has been implicated in the disruption of cellular metabolic processes, including oxidative phosphorylation and lipid metabolism .
Molecular Mechanism
At the molecular level, 2,2’,4,4’,6,6’-Hexabromobiphenyl exerts its effects through various binding interactions and enzyme modulations. The compound’s binding to the AhR receptor triggers a cascade of molecular events, including the translocation of the receptor-ligand complex to the nucleus, where it binds to xenobiotic response elements (XREs) in the promoter regions of target genes . This binding results in the transcriptional activation of genes encoding phase I and II detoxification enzymes, such as CYP1A1 . Additionally, 2,2’,4,4’,6,6’-Hexabromobiphenyl can inhibit or activate other enzymes involved in cellular metabolism, further contributing to its biochemical effects .
Temporal Effects in Laboratory Settings
The temporal effects of 2,2’,4,4’,6,6’-Hexabromobiphenyl in laboratory settings have been extensively studied to understand its stability, degradation, and long-term impacts on cellular function. Studies have shown that 2,2’,4,4’,6,6’-Hexabromobiphenyl is relatively stable and resistant to degradation, which contributes to its persistence in the environment . Over time, the compound can accumulate in biological tissues, leading to prolonged exposure and potential toxic effects . Long-term studies have also indicated that chronic exposure to 2,2’,4,4’,6,6’-Hexabromobiphenyl can result in significant alterations in cellular function, including changes in gene expression and metabolic activity .
Dosage Effects in Animal Models
The effects of 2,2’,4,4’,6,6’-Hexabromobiphenyl vary with different dosages in animal models. At low doses, the compound may induce mild biochemical and cellular changes, while higher doses can lead to more severe toxic effects . Threshold effects have been observed, where a certain dosage level must be reached before significant biological responses are elicited . High doses of 2,2’,4,4’,6,6’-Hexabromobiphenyl have been associated with adverse effects, including hepatotoxicity, neurotoxicity, and reproductive toxicity .
Metabolic Pathways
2,2’,4,4’,6,6’-Hexabromobiphenyl is involved in several metabolic pathways, primarily those related to xenobiotic metabolism. The compound is metabolized by cytochrome P450 enzymes, particularly CYP1A1, which catalyze its biotransformation into more water-soluble metabolites that can be excreted from the body . This metabolic process involves phase I reactions, such as hydroxylation, followed by phase II reactions, including conjugation with glucuronic acid or sulfate . These metabolic pathways are essential for the detoxification and elimination of 2,2’,4,4’,6,6’-Hexabromobiphenyl from the body .
Transport and Distribution
The transport and distribution of 2,2’,4,4’,6,6’-Hexabromobiphenyl within cells and tissues are influenced by its physicochemical properties and interactions with transport proteins. The compound can be transported across cell membranes via passive diffusion or facilitated by specific transporters . Once inside the cell, 2,2’,4,4’,6,6’-Hexabromobiphenyl can bind to intracellular proteins, such as cytosolic receptors and enzymes, which affect its localization and accumulation . The distribution of 2,2’,4,4’,6,6’-Hexabromobiphenyl in tissues is also determined by its lipophilicity, allowing it to accumulate in lipid-rich tissues .
Subcellular Localization
The subcellular localization of 2,2’,4,4’,6,6’-Hexabromobiphenyl is critical for understanding its activity and function. The compound is primarily localized in the cytoplasm, where it interacts with various cytosolic proteins and enzymes . Additionally, 2,2’,4,4’,6,6’-Hexabromobiphenyl can be transported to the nucleus upon binding to the AhR receptor, where it influences gene expression . The subcellular distribution of 2,2’,4,4’,6,6’-Hexabromobiphenyl is also affected by post-translational modifications and targeting signals that direct it to specific cellular compartments .
Preparation Methods
The synthesis of 2,2’,4,4’,6,6’-Hexabromobiphenyl typically involves the bromination of biphenyl compounds. One common synthetic route is the bromination of 1,3,5-tribromobenzene . The reaction conditions often include the use of bromine or bromine-containing reagents under controlled temperatures to ensure selective bromination at the desired positions on the biphenyl ring . Industrial production methods have historically involved large-scale bromination processes, but due to its environmental impact, production has been significantly reduced .
Chemical Reactions Analysis
2,2’,4,4’,6,6’-Hexabromobiphenyl undergoes several types of chemical reactions, including:
Oxidation: This compound can be oxidized under specific conditions, leading to the formation of various brominated by-products.
Reduction: Reduction reactions can remove bromine atoms, resulting in less brominated biphenyls.
Substitution: Halogen exchange reactions can occur, where bromine atoms are replaced by other halogens or functional groups.
Common reagents used in these reactions include strong oxidizing agents like potassium permanganate for oxidation, reducing agents like sodium borohydride for reduction, and halogenating agents for substitution reactions . The major products formed depend on the specific reaction conditions and reagents used.
Scientific Research Applications
2,2’,4,4’,6,6’-Hexabromobiphenyl has been studied extensively for its environmental and biological impacts. In chemistry, it serves as a model compound for studying the behavior of polybrominated biphenyls in various environments . In biology and medicine, research has focused on its toxicological effects, including its potential as a carcinogen and its impact on endocrine systems . Industrially, it was used as a flame retardant in plastics and electronic devices, although its use has been largely discontinued due to environmental concerns .
Comparison with Similar Compounds
2,2’,4,4’,6,6’-Hexabromobiphenyl is part of a broader class of polybrominated biphenyls, which include compounds like 2,2’,4,4’,5,5’-Hexabromobiphenyl and 2,2’,3,3’,4,4’-Hexabromobiphenyl . Compared to these similar compounds, 2,2’,4,4’,6,6’-Hexabromobiphenyl is notable for its specific bromination pattern, which influences its chemical reactivity and environmental behavior . Its high degree of bromination makes it particularly persistent and bioaccumulative, distinguishing it from less brominated analogs .
Properties
IUPAC Name |
1,3,5-tribromo-2-(2,4,6-tribromophenyl)benzene | |
---|---|---|
Source | PubChem | |
URL | https://pubchem.ncbi.nlm.nih.gov | |
Description | Data deposited in or computed by PubChem | |
InChI |
InChI=1S/C12H4Br6/c13-5-1-7(15)11(8(16)2-5)12-9(17)3-6(14)4-10(12)18/h1-4H | |
Source | PubChem | |
URL | https://pubchem.ncbi.nlm.nih.gov | |
Description | Data deposited in or computed by PubChem | |
InChI Key |
LNFYSRMCCKKDEH-UHFFFAOYSA-N | |
Source | PubChem | |
URL | https://pubchem.ncbi.nlm.nih.gov | |
Description | Data deposited in or computed by PubChem | |
Canonical SMILES |
C1=C(C=C(C(=C1Br)C2=C(C=C(C=C2Br)Br)Br)Br)Br | |
Source | PubChem | |
URL | https://pubchem.ncbi.nlm.nih.gov | |
Description | Data deposited in or computed by PubChem | |
Molecular Formula |
C12H4Br6 | |
Source | PubChem | |
URL | https://pubchem.ncbi.nlm.nih.gov | |
Description | Data deposited in or computed by PubChem | |
DSSTOX Substance ID |
DTXSID4074772 | |
Record name | PBB 155 | |
Source | EPA DSSTox | |
URL | https://comptox.epa.gov/dashboard/DTXSID4074772 | |
Description | DSSTox provides a high quality public chemistry resource for supporting improved predictive toxicology. | |
Molecular Weight |
627.6 g/mol | |
Source | PubChem | |
URL | https://pubchem.ncbi.nlm.nih.gov | |
Description | Data deposited in or computed by PubChem | |
CAS No. |
59261-08-4 | |
Record name | 2,2',4,4',6,6'-Hexabromobiphenyl | |
Source | ChemIDplus | |
URL | https://pubchem.ncbi.nlm.nih.gov/substance/?source=chemidplus&sourceid=0059261084 | |
Description | ChemIDplus is a free, web search system that provides access to the structure and nomenclature authority files used for the identification of chemical substances cited in National Library of Medicine (NLM) databases, including the TOXNET system. | |
Record name | 59261-08-4 | |
Source | DTP/NCI | |
URL | https://dtp.cancer.gov/dtpstandard/servlet/dwindex?searchtype=NSC&outputformat=html&searchlist=157312 | |
Description | The NCI Development Therapeutics Program (DTP) provides services and resources to the academic and private-sector research communities worldwide to facilitate the discovery and development of new cancer therapeutic agents. | |
Explanation | Unless otherwise indicated, all text within NCI products is free of copyright and may be reused without our permission. Credit the National Cancer Institute as the source. | |
Record name | PBB 155 | |
Source | EPA DSSTox | |
URL | https://comptox.epa.gov/dashboard/DTXSID4074772 | |
Description | DSSTox provides a high quality public chemistry resource for supporting improved predictive toxicology. | |
Record name | 2,2',4,4',6,6'-HEXABROMOBIPHENYL | |
Source | FDA Global Substance Registration System (GSRS) | |
URL | https://gsrs.ncats.nih.gov/ginas/app/beta/substances/642OIA2442 | |
Description | The FDA Global Substance Registration System (GSRS) enables the efficient and accurate exchange of information on what substances are in regulated products. Instead of relying on names, which vary across regulatory domains, countries, and regions, the GSRS knowledge base makes it possible for substances to be defined by standardized, scientific descriptions. | |
Explanation | Unless otherwise noted, the contents of the FDA website (www.fda.gov), both text and graphics, are not copyrighted. They are in the public domain and may be republished, reprinted and otherwise used freely by anyone without the need to obtain permission from FDA. Credit to the U.S. Food and Drug Administration as the source is appreciated but not required. | |
Retrosynthesis Analysis
AI-Powered Synthesis Planning: Our tool employs the Template_relevance Pistachio, Template_relevance Bkms_metabolic, Template_relevance Pistachio_ringbreaker, Template_relevance Reaxys, Template_relevance Reaxys_biocatalysis model, leveraging a vast database of chemical reactions to predict feasible synthetic routes.
One-Step Synthesis Focus: Specifically designed for one-step synthesis, it provides concise and direct routes for your target compounds, streamlining the synthesis process.
Accurate Predictions: Utilizing the extensive PISTACHIO, BKMS_METABOLIC, PISTACHIO_RINGBREAKER, REAXYS, REAXYS_BIOCATALYSIS database, our tool offers high-accuracy predictions, reflecting the latest in chemical research and data.
Strategy Settings
Precursor scoring | Relevance Heuristic |
---|---|
Min. plausibility | 0.01 |
Model | Template_relevance |
Template Set | Pistachio/Bkms_metabolic/Pistachio_ringbreaker/Reaxys/Reaxys_biocatalysis |
Top-N result to add to graph | 6 |
Feasible Synthetic Routes
Q1: What is the crystal structure of 2,2',4,4',6,6'-Hexabromobiphenyl and how does its structure relate to other similar compounds?
A1: this compound crystallizes in the orthorhombic space group P nca with unit cell dimensions of a = 14.48(1) Å, b = 12.71(1) Å, and c = 8.534(7) Å. [] The structure was determined to have a residual (R) value of 0.044 for 693 observed reflections. Notably, the dihedral angle (θ) between the two phenyl rings, influenced by the steric interactions of the bromine substituents, is 83.0°. [] This angle is comparable to the 81.7° observed in the structurally similar compound, 2,2',6,6'-tetrachlorobiphenyl. [] The study highlights that steric interactions are not the only factor determining the solid-state geometry of these compounds. Interestingly, this compound and 2,2',6,6'-tetrachlorobiphenyl exhibit pseudo-isostructural characteristics, further emphasizing the influence of halogen substitution on the structural arrangement. []
Q2: Can you describe an analytical method used to determine the presence of this compound in a complex matrix like a food package?
A2: While the provided research [] focuses on structural analysis, a separate study [] outlines a method for determining various additives, including fire retardants like this compound, in plastic food packaging. This method employs ultrasonic extraction followed by analysis using Ultra Performance Liquid Chromatography coupled with a Photodiode Array Detector (UPLC-PDA). [] This technique is particularly suitable for complex matrices due to its high sensitivity and selectivity. The study highlights that this method achieves a limit of detection (LOD) ranging from 0.01 to 0.55 µg/mL for the analyzed additives, showcasing its ability to detect trace amounts of these compounds. [] The researchers achieved good accuracy with average spiked recoveries exceeding 70.24% for most analytes, including this compound. [] This method offers a robust approach to monitoring the presence of fire retardants like this compound in materials intended for food contact, ensuring consumer safety.
Disclaimer and Information on In-Vitro Research Products
Please be aware that all articles and product information presented on BenchChem are intended solely for informational purposes. The products available for purchase on BenchChem are specifically designed for in-vitro studies, which are conducted outside of living organisms. In-vitro studies, derived from the Latin term "in glass," involve experiments performed in controlled laboratory settings using cells or tissues. It is important to note that these products are not categorized as medicines or drugs, and they have not received approval from the FDA for the prevention, treatment, or cure of any medical condition, ailment, or disease. We must emphasize that any form of bodily introduction of these products into humans or animals is strictly prohibited by law. It is essential to adhere to these guidelines to ensure compliance with legal and ethical standards in research and experimentation.