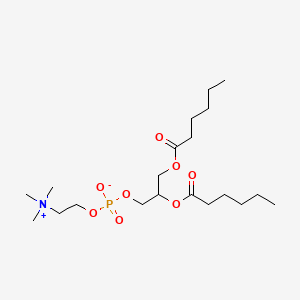
Dihexanoyl-lecithin
Overview
Description
Dihexanoyl-lecithin is a synthetic phospholipid, structurally similar to natural lecithins found in biological membranes. It consists of a glycerol backbone esterified with two hexanoic acid molecules and a phosphocholine head group. This compound is known for its amphiphilic properties, making it useful in various biochemical and biophysical studies .
Preparation Methods
Synthetic Routes and Reaction Conditions: Dihexanoyl-lecithin can be synthesized through esterification of glycerol with hexanoic acid, followed by phosphorylation to introduce the phosphocholine head group. The reaction typically involves:
Esterification: Glycerol reacts with hexanoic acid in the presence of a catalyst such as sulfuric acid.
Phosphorylation: The resulting dihexanoyl-glycerol is then phosphorylated using phosphocholine chloride under basic conditions.
Industrial Production Methods: Industrial production of this compound involves similar steps but on a larger scale, with optimized reaction conditions to ensure high yield and purity. The process includes:
Purification: Using techniques like chromatography to isolate the desired product.
Quality Control: Ensuring the final product meets industry standards for use in research and applications.
Chemical Reactions Analysis
Types of Reactions: Dihexanoyl-lecithin undergoes various chemical reactions, including:
Hydrolysis: Enzymatic cleavage by phospholipase A2, resulting in the release of fatty acids and glycerophosphocholine.
Oxidation: Reaction with oxidizing agents can lead to the formation of peroxides and other oxidative products.
Common Reagents and Conditions:
Hydrolysis: Catalyzed by enzymes like phospholipase A2 under physiological conditions.
Oxidation: Typically involves oxidizing agents such as hydrogen peroxide or molecular oxygen.
Major Products:
Hydrolysis: Produces hexanoic acid and glycerophosphocholine.
Oxidation: Results in various oxidative derivatives depending on the specific conditions and reagents used.
Scientific Research Applications
Dihexanoyl-lecithin has a wide range of applications in scientific research:
Biochemistry: Used to study membrane-associated processes and protein-lipid interactions due to its ability to form micelles and bilayers.
Biophysics: Employed in the formation of bicelles, which are useful for NMR studies of membrane proteins.
Industry: Utilized as an emulsifier in food and cosmetic products due to its amphiphilic nature.
Mechanism of Action
Dihexanoyl-lecithin exerts its effects primarily through its ability to integrate into lipid bilayers and form micelles. This integration can alter membrane fluidity and permeability, affecting various cellular processes. The compound targets membrane proteins and can modulate their activity by changing the local lipid environment .
Comparison with Similar Compounds
Diheptanoyl-lecithin: Another short-chain lecithin with similar properties but different micellar weight distributions.
Dimyristoyl phosphatidylcholine (DMPC): A long-chain phospholipid used in similar applications but with different physical properties.
Uniqueness: Dihexanoyl-lecithin is unique due to its short-chain fatty acids, which confer distinct micellar properties and make it particularly useful for studying membrane dynamics and protein-lipid interactions .
Properties
IUPAC Name |
2,3-di(hexanoyloxy)propyl 2-(trimethylazaniumyl)ethyl phosphate | |
---|---|---|
Source | PubChem | |
URL | https://pubchem.ncbi.nlm.nih.gov | |
Description | Data deposited in or computed by PubChem | |
InChI |
InChI=1S/C20H40NO8P/c1-6-8-10-12-19(22)26-16-18(29-20(23)13-11-9-7-2)17-28-30(24,25)27-15-14-21(3,4)5/h18H,6-17H2,1-5H3 | |
Source | PubChem | |
URL | https://pubchem.ncbi.nlm.nih.gov | |
Description | Data deposited in or computed by PubChem | |
InChI Key |
DVZARZBAWHITHR-UHFFFAOYSA-N | |
Source | PubChem | |
URL | https://pubchem.ncbi.nlm.nih.gov | |
Description | Data deposited in or computed by PubChem | |
Canonical SMILES |
CCCCCC(=O)OCC(COP(=O)([O-])OCC[N+](C)(C)C)OC(=O)CCCCC | |
Source | PubChem | |
URL | https://pubchem.ncbi.nlm.nih.gov | |
Description | Data deposited in or computed by PubChem | |
Molecular Formula |
C20H40NO8P | |
Source | PubChem | |
URL | https://pubchem.ncbi.nlm.nih.gov | |
Description | Data deposited in or computed by PubChem | |
DSSTOX Substance ID |
DTXSID80968679 | |
Record name | 2,3-Bis(hexanoyloxy)propyl 2-(trimethylazaniumyl)ethyl phosphate | |
Source | EPA DSSTox | |
URL | https://comptox.epa.gov/dashboard/DTXSID80968679 | |
Description | DSSTox provides a high quality public chemistry resource for supporting improved predictive toxicology. | |
Molecular Weight |
453.5 g/mol | |
Source | PubChem | |
URL | https://pubchem.ncbi.nlm.nih.gov | |
Description | Data deposited in or computed by PubChem | |
CAS No. |
53892-41-4 | |
Record name | 1,2-Hexanoylphosphatidylcholine | |
Source | ChemIDplus | |
URL | https://pubchem.ncbi.nlm.nih.gov/substance/?source=chemidplus&sourceid=0053892414 | |
Description | ChemIDplus is a free, web search system that provides access to the structure and nomenclature authority files used for the identification of chemical substances cited in National Library of Medicine (NLM) databases, including the TOXNET system. | |
Record name | 2,3-Bis(hexanoyloxy)propyl 2-(trimethylazaniumyl)ethyl phosphate | |
Source | EPA DSSTox | |
URL | https://comptox.epa.gov/dashboard/DTXSID80968679 | |
Description | DSSTox provides a high quality public chemistry resource for supporting improved predictive toxicology. | |
Retrosynthesis Analysis
AI-Powered Synthesis Planning: Our tool employs the Template_relevance Pistachio, Template_relevance Bkms_metabolic, Template_relevance Pistachio_ringbreaker, Template_relevance Reaxys, Template_relevance Reaxys_biocatalysis model, leveraging a vast database of chemical reactions to predict feasible synthetic routes.
One-Step Synthesis Focus: Specifically designed for one-step synthesis, it provides concise and direct routes for your target compounds, streamlining the synthesis process.
Accurate Predictions: Utilizing the extensive PISTACHIO, BKMS_METABOLIC, PISTACHIO_RINGBREAKER, REAXYS, REAXYS_BIOCATALYSIS database, our tool offers high-accuracy predictions, reflecting the latest in chemical research and data.
Strategy Settings
Precursor scoring | Relevance Heuristic |
---|---|
Min. plausibility | 0.01 |
Model | Template_relevance |
Template Set | Pistachio/Bkms_metabolic/Pistachio_ringbreaker/Reaxys/Reaxys_biocatalysis |
Top-N result to add to graph | 6 |
Feasible Synthetic Routes
Q1: What is the role of Dihexanoyl-lecithin in studying Phospholipase A2 (PLA2) enzymes?
A1: this compound is a short-chain phospholipid often employed as a substrate to study the activity of Phospholipase A2 (PLA2) enzymes. [, ] PLA2 enzymes catalyze the hydrolysis of phospholipids at the sn-2 position, releasing a fatty acid and a lysophospholipid. [] Due to its relatively simple structure and solubility in aqueous solutions, this compound provides a convenient model substrate for investigating the kinetics, substrate specificity, and mechanism of action of PLA2 enzymes.
Q2: Can you explain the significance of using this compound as a substrate in a continuous assay for Phospholipase A activity?
A3: this compound serves as an ideal substrate in a continuous assay for Phospholipase A activity due to the distinct properties of its hydrolysis products. [] When this compound is hydrolyzed by Phospholipase A, it releases hexanoic acid. This fatty acid is readily detectable using a continuous titrimetric method, allowing researchers to monitor the reaction progress in real-time and accurately determine the enzyme's activity. [] This method offers several advantages, including increased sensitivity, simplified procedures, and the ability to study enzyme kinetics more effectively.
Disclaimer and Information on In-Vitro Research Products
Please be aware that all articles and product information presented on BenchChem are intended solely for informational purposes. The products available for purchase on BenchChem are specifically designed for in-vitro studies, which are conducted outside of living organisms. In-vitro studies, derived from the Latin term "in glass," involve experiments performed in controlled laboratory settings using cells or tissues. It is important to note that these products are not categorized as medicines or drugs, and they have not received approval from the FDA for the prevention, treatment, or cure of any medical condition, ailment, or disease. We must emphasize that any form of bodily introduction of these products into humans or animals is strictly prohibited by law. It is essential to adhere to these guidelines to ensure compliance with legal and ethical standards in research and experimentation.