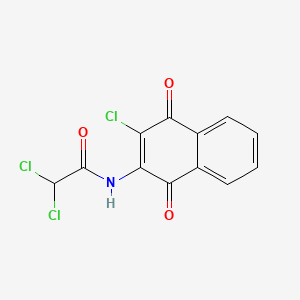
Quinonamid
Overview
Description
Mechanism of Action
Target of Action
Quinonamid, like other quinolones, primarily targets bacterial enzymes such as gyrase and topoisomerase IV . These enzymes play a crucial role in bacterial DNA replication, making them ideal targets for antibacterial agents .
Mode of Action
This compound acts by converting its targets, gyrase and topoisomerase IV, into toxic enzymes that fragment the bacterial chromosome . This interaction disrupts the normal functioning of these enzymes, leading to the inhibition of bacterial DNA replication and ultimately, bacterial death .
Biochemical Pathways
It is known that quinolones interfere with the dna supercoiling process, which is essential for dna replication and transcription . By inhibiting this process, this compound disrupts the normal cellular functions of bacteria, leading to their death .
Pharmacokinetics
Quinolones, in general, are known for their excellent tissue penetration and concentrations, as reflected in their particularly large apparent volumes of distribution . These properties contribute to their effectiveness as antibacterial agents .
Result of Action
The primary result of this compound’s action is the death of bacterial cells. By inhibiting the function of key bacterial enzymes, this compound disrupts DNA replication, leading to cell death . This makes it an effective antibacterial agent.
Action Environment
The action, efficacy, and stability of this compound can be influenced by various environmental factors. It’s important to note that the effectiveness of many antibacterial agents can be influenced by factors such as pH, temperature, and the presence of other substances .
Biochemical Analysis
Biochemical Properties
Quinonamid plays a significant role in biochemical reactions, particularly in inhibiting photosynthetic oxygen production in algal suspensions . It acts as a photosynthetic electron transport blocker, disrupting the normal electron flow within the photosystem. This compound interacts with various enzymes and proteins involved in the photosynthetic process, such as photosystem II, where it binds to the D1 protein and inhibits the electron transfer chain . This interaction leads to the generation of reactive oxygen species, causing oxidative stress and cellular damage in algae.
Cellular Effects
This compound exerts various effects on different types of cells and cellular processes. In algal cells, it disrupts photosynthesis by inhibiting the electron transport chain, leading to reduced ATP production and impaired cellular metabolism . This inhibition affects cell signaling pathways, particularly those involved in energy production and stress responses. This compound also influences gene expression by upregulating stress-responsive genes and downregulating genes involved in photosynthesis . In mammalian cells, this compound has been shown to induce oxidative stress and apoptosis, affecting cell viability and function .
Molecular Mechanism
The molecular mechanism of this compound involves its binding interactions with biomolecules and its ability to inhibit enzyme activity. This compound binds to the D1 protein in photosystem II, blocking the electron transfer chain and leading to the accumulation of reactive oxygen species . This oxidative stress damages cellular components, including lipids, proteins, and DNA, ultimately resulting in cell death. This compound also affects gene expression by modulating transcription factors and signaling pathways involved in stress responses .
Temporal Effects in Laboratory Settings
In laboratory settings, the effects of this compound change over time due to its stability and degradation. This compound is relatively stable under standard laboratory conditions, but it can degrade over time, leading to reduced efficacy . Long-term exposure to this compound in in vitro studies has shown persistent oxidative stress and cellular damage, affecting cell viability and function . In in vivo studies, prolonged exposure to this compound has been associated with chronic toxicity and adverse effects on organ function .
Dosage Effects in Animal Models
The effects of this compound vary with different dosages in animal models. At low doses, this compound has been shown to induce mild oxidative stress and cellular damage . At higher doses, this compound can cause significant toxicity, leading to severe oxidative stress, apoptosis, and organ damage . Threshold effects have been observed, where a certain dosage level triggers a marked increase in adverse effects. Toxicity studies in animal models have highlighted the potential risks associated with high-dose exposure to this compound .
Metabolic Pathways
This compound is involved in various metabolic pathways, particularly those related to oxidative stress and detoxification. It interacts with enzymes such as cytochrome P450, which metabolizes this compound into reactive intermediates . These intermediates can further react with cellular components, leading to oxidative damage. This compound also affects metabolic flux by altering the levels of key metabolites involved in energy production and stress responses .
Transport and Distribution
Within cells and tissues, this compound is transported and distributed through various mechanisms. It can diffuse across cell membranes and accumulate in specific cellular compartments . This compound interacts with transporters and binding proteins that facilitate its movement within cells. Its localization and accumulation can affect its activity and function, particularly in target tissues such as algae and mammalian cells .
Subcellular Localization
This compound exhibits specific subcellular localization, which influences its activity and function. It primarily localizes to the chloroplasts in algal cells, where it exerts its inhibitory effects on photosynthesis . In mammalian cells, this compound can localize to the mitochondria and induce oxidative stress, affecting mitochondrial function and energy production . Targeting signals and post-translational modifications may direct this compound to specific compartments or organelles, enhancing its efficacy and specificity .
Preparation Methods
Quinonamid is synthesized through the acylation reaction of 2-amino-3-chloro-1,4-naphthoquinone with 2,2-dichloroacetyl chloride . The reaction typically occurs in the presence of a base, such as pyridine, to facilitate the acylation process. The reaction conditions include maintaining a temperature range of 0-5°C to control the exothermic nature of the reaction .
Industrial production of this compound involves similar synthetic routes but on a larger scale. The process includes the purification of the final product through recrystallization from suitable solvents to achieve the desired purity and yield .
Chemical Reactions Analysis
Quinonamid undergoes various chemical reactions, including:
Oxidation: this compound can be oxidized to form quinone derivatives. Common oxidizing agents include potassium permanganate and hydrogen peroxide.
Reduction: Reduction of this compound can lead to the formation of hydroquinone derivatives. Reducing agents such as sodium borohydride or lithium aluminum hydride are typically used.
Substitution: this compound can undergo nucleophilic substitution reactions, particularly at the chlorine atoms. Reagents such as sodium methoxide or potassium tert-butoxide are commonly used for these reactions.
The major products formed from these reactions include various substituted quinones and hydroquinones, which have significant applications in organic synthesis and medicinal chemistry .
Scientific Research Applications
Quinonamid has a wide range of scientific research applications:
Comparison with Similar Compounds
Quinonamid is structurally similar to other quinone derivatives, such as:
Benzoquinone: A simple quinone with a similar electron-accepting capability but lacks the chlorine substituents present in this compound.
Naphthoquinone: Shares the naphthalene ring structure with this compound but differs in the substitution pattern.
Anthraquinone: Contains an additional aromatic ring compared to this compound, leading to different chemical properties and applications.
This compound’s uniqueness lies in its specific substitution pattern, which imparts distinct chemical reactivity and biological activity compared to other quinone derivatives .
Properties
IUPAC Name |
2,2-dichloro-N-(3-chloro-1,4-dioxonaphthalen-2-yl)acetamide | |
---|---|---|
Source | PubChem | |
URL | https://pubchem.ncbi.nlm.nih.gov | |
Description | Data deposited in or computed by PubChem | |
InChI |
InChI=1S/C12H6Cl3NO3/c13-7-8(16-12(19)11(14)15)10(18)6-4-2-1-3-5(6)9(7)17/h1-4,11H,(H,16,19) | |
Source | PubChem | |
URL | https://pubchem.ncbi.nlm.nih.gov | |
Description | Data deposited in or computed by PubChem | |
InChI Key |
ZIEWAMOXCOLNSJ-UHFFFAOYSA-N | |
Source | PubChem | |
URL | https://pubchem.ncbi.nlm.nih.gov | |
Description | Data deposited in or computed by PubChem | |
Canonical SMILES |
C1=CC=C2C(=C1)C(=O)C(=C(C2=O)Cl)NC(=O)C(Cl)Cl | |
Source | PubChem | |
URL | https://pubchem.ncbi.nlm.nih.gov | |
Description | Data deposited in or computed by PubChem | |
Molecular Formula |
C12H6Cl3NO3 | |
Source | PubChem | |
URL | https://pubchem.ncbi.nlm.nih.gov | |
Description | Data deposited in or computed by PubChem | |
DSSTOX Substance ID |
DTXSID2058047 | |
Record name | Quinonamid | |
Source | EPA DSSTox | |
URL | https://comptox.epa.gov/dashboard/DTXSID2058047 | |
Description | DSSTox provides a high quality public chemistry resource for supporting improved predictive toxicology. | |
Molecular Weight |
318.5 g/mol | |
Source | PubChem | |
URL | https://pubchem.ncbi.nlm.nih.gov | |
Description | Data deposited in or computed by PubChem | |
CAS No. |
27541-88-4 | |
Record name | Quinonamid | |
Source | CAS Common Chemistry | |
URL | https://commonchemistry.cas.org/detail?cas_rn=27541-88-4 | |
Description | CAS Common Chemistry is an open community resource for accessing chemical information. Nearly 500,000 chemical substances from CAS REGISTRY cover areas of community interest, including common and frequently regulated chemicals, and those relevant to high school and undergraduate chemistry classes. This chemical information, curated by our expert scientists, is provided in alignment with our mission as a division of the American Chemical Society. | |
Explanation | The data from CAS Common Chemistry is provided under a CC-BY-NC 4.0 license, unless otherwise stated. | |
Record name | Quinonamid [ISO] | |
Source | ChemIDplus | |
URL | https://pubchem.ncbi.nlm.nih.gov/substance/?source=chemidplus&sourceid=0027541884 | |
Description | ChemIDplus is a free, web search system that provides access to the structure and nomenclature authority files used for the identification of chemical substances cited in National Library of Medicine (NLM) databases, including the TOXNET system. | |
Record name | Quinonamid | |
Source | EPA DSSTox | |
URL | https://comptox.epa.gov/dashboard/DTXSID2058047 | |
Description | DSSTox provides a high quality public chemistry resource for supporting improved predictive toxicology. | |
Record name | 2,2-dichloro-N-(3-chloro-1,4-naphthoquinon-2-yl)acetamide | |
Source | European Chemicals Agency (ECHA) | |
URL | https://echa.europa.eu/substance-information/-/substanceinfo/100.044.091 | |
Description | The European Chemicals Agency (ECHA) is an agency of the European Union which is the driving force among regulatory authorities in implementing the EU's groundbreaking chemicals legislation for the benefit of human health and the environment as well as for innovation and competitiveness. | |
Explanation | Use of the information, documents and data from the ECHA website is subject to the terms and conditions of this Legal Notice, and subject to other binding limitations provided for under applicable law, the information, documents and data made available on the ECHA website may be reproduced, distributed and/or used, totally or in part, for non-commercial purposes provided that ECHA is acknowledged as the source: "Source: European Chemicals Agency, http://echa.europa.eu/". Such acknowledgement must be included in each copy of the material. ECHA permits and encourages organisations and individuals to create links to the ECHA website under the following cumulative conditions: Links can only be made to webpages that provide a link to the Legal Notice page. | |
Record name | QUINONAMID | |
Source | FDA Global Substance Registration System (GSRS) | |
URL | https://gsrs.ncats.nih.gov/ginas/app/beta/substances/618QV11Q2U | |
Description | The FDA Global Substance Registration System (GSRS) enables the efficient and accurate exchange of information on what substances are in regulated products. Instead of relying on names, which vary across regulatory domains, countries, and regions, the GSRS knowledge base makes it possible for substances to be defined by standardized, scientific descriptions. | |
Explanation | Unless otherwise noted, the contents of the FDA website (www.fda.gov), both text and graphics, are not copyrighted. They are in the public domain and may be republished, reprinted and otherwise used freely by anyone without the need to obtain permission from FDA. Credit to the U.S. Food and Drug Administration as the source is appreciated but not required. | |
Retrosynthesis Analysis
AI-Powered Synthesis Planning: Our tool employs the Template_relevance Pistachio, Template_relevance Bkms_metabolic, Template_relevance Pistachio_ringbreaker, Template_relevance Reaxys, Template_relevance Reaxys_biocatalysis model, leveraging a vast database of chemical reactions to predict feasible synthetic routes.
One-Step Synthesis Focus: Specifically designed for one-step synthesis, it provides concise and direct routes for your target compounds, streamlining the synthesis process.
Accurate Predictions: Utilizing the extensive PISTACHIO, BKMS_METABOLIC, PISTACHIO_RINGBREAKER, REAXYS, REAXYS_BIOCATALYSIS database, our tool offers high-accuracy predictions, reflecting the latest in chemical research and data.
Strategy Settings
Precursor scoring | Relevance Heuristic |
---|---|
Min. plausibility | 0.01 |
Model | Template_relevance |
Template Set | Pistachio/Bkms_metabolic/Pistachio_ringbreaker/Reaxys/Reaxys_biocatalysis |
Top-N result to add to graph | 6 |
Feasible Synthetic Routes
Q1: How does Quinonamid interact with its target and what are the downstream effects?
A: this compound specifically targets the photosynthetic electron transport chain in Photosystem II (PSII) of algae and plants. [] It acts as an electron transport blocker, disrupting the flow of electrons and effectively inhibiting the production of oxygen from water during photosynthesis. [] This ultimately leads to the suppression of algal growth and can have detrimental effects on plant life.
Q2: What is known about the Structure-Activity Relationship (SAR) of this compound and its analogs?
A: The research paper compares this compound with a closely related compound, 2-amino-3-chloro-1,4-naphthoquinone (Hoe 17399). [] This comparison suggests that the presence of the dichloroacetyl group in this compound contributes to its stronger inhibitory effect on photosynthetic electron transport compared to Hoe 17399. [] This highlights the importance of specific structural features in modulating the potency of these naphthoquinone derivatives. Further research exploring modifications to the this compound structure could reveal valuable insights into optimizing its inhibitory activity for specific applications.
Disclaimer and Information on In-Vitro Research Products
Please be aware that all articles and product information presented on BenchChem are intended solely for informational purposes. The products available for purchase on BenchChem are specifically designed for in-vitro studies, which are conducted outside of living organisms. In-vitro studies, derived from the Latin term "in glass," involve experiments performed in controlled laboratory settings using cells or tissues. It is important to note that these products are not categorized as medicines or drugs, and they have not received approval from the FDA for the prevention, treatment, or cure of any medical condition, ailment, or disease. We must emphasize that any form of bodily introduction of these products into humans or animals is strictly prohibited by law. It is essential to adhere to these guidelines to ensure compliance with legal and ethical standards in research and experimentation.