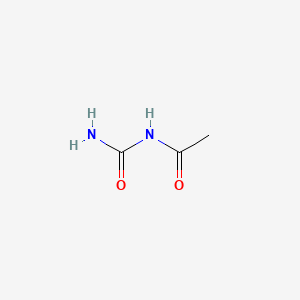
Acetylurea
Overview
Description
Acetylurea, also known as N-acetylurea, is a chemical compound with the molecular formula C3H6N2O2. It is a derivative of urea, where one of the hydrogen atoms in the urea molecule is replaced by an acetyl group. This compound is known for its applications in various fields, including pharmaceuticals and chemical research.
Mechanism of Action
Target of Action
Acetylurea, also known as phenylthis compound, is an anticonvulsant of the ureide (this compound) class . The primary targets of this compound are the organic anion transporters (OAT1 and OAT3) and the monocarboxylate transporter type 1 (MCT1) . These transporters are responsible for the uptake of this compound into cells .
Mode of Action
This compound interacts with its targets by switching the uptake of leucine into cells from the L-type amino acid transporter (LAT1) used by leucine to OAT1, OAT3, and MCT1 . This switch allows this compound to bypass LAT1, which is the rate-limiting step in the activation of leucine-mediated signaling and metabolic processes inside cells .
Biochemical Pathways
The this compound portion of phenacemide and ethylphenacemide folds back upon itself to form a six-atom ring with the open end closed by an intramolecular hydrogen bond between a hydrogen atom on the terminal nitrogen and a carbonyl oxygen . This process is part of the acetyl CoA pathway, which is the only pathway of CO2 fixation coupled to energy storage .
Pharmacokinetics
Phenacemide, a type of this compound, is rapidly and completely absorbed from the small intestine when orally administered to mice . The pharmacokinetic factors may play a major role in its mechanism of action and efficacy as a drug .
Result of Action
The molecular and cellular effects of this compound’s action are primarily seen in its ability to bypass LAT1, thereby enhancing leucine-mediated signaling and metabolic processes inside cells . This can have profound effects on the pharmacological activity of the compound, making drugs more effective by enhancing their pharmacokinetic or pharmacodynamic properties .
Biochemical Analysis
Biochemical Properties
Acetylurea plays a significant role in biochemical reactions, particularly in the synthesis of pharmaceutical compounds. It interacts with various enzymes and proteins, including those involved in the synthesis of barbiturates and antiepileptic drugs . The nature of these interactions often involves the formation of stable complexes that facilitate the desired chemical transformations. For instance, this compound can react with acid halides or anhydrides to form more complex ureides .
Cellular Effects
This compound influences various cellular processes, including cell signaling pathways, gene expression, and cellular metabolism. It has been observed to affect the function of neurons, which is why it is used in the synthesis of anticonvulsant drugs . The compound can modulate the activity of neurotransmitters and ion channels, thereby impacting neuronal excitability and synaptic transmission . Additionally, this compound may influence the expression of genes involved in metabolic pathways, further affecting cellular metabolism .
Molecular Mechanism
The molecular mechanism of this compound involves its interaction with specific biomolecules, leading to enzyme inhibition or activation and changes in gene expression. This compound can bind to enzymes such as acetyl-CoA carboxylase, inhibiting its activity and thereby affecting fatty acid metabolism . This inhibition can lead to a decrease in the synthesis of fatty acids, which has implications for cellular energy balance and metabolic regulation .
Temporal Effects in Laboratory Settings
In laboratory settings, the effects of this compound can change over time due to its stability and degradation. Studies have shown that this compound is relatively stable under standard laboratory conditions, but it can degrade over extended periods or under extreme conditions . Long-term exposure to this compound has been observed to cause changes in cellular function, including alterations in metabolic activity and gene expression .
Dosage Effects in Animal Models
The effects of this compound vary with different dosages in animal models. At low doses, this compound can have therapeutic effects, such as anticonvulsant activity . At high doses, it can cause toxic or adverse effects, including neurotoxicity and hepatotoxicity . Threshold effects have been observed, where the compound exhibits a dose-dependent response, with higher doses leading to more pronounced effects .
Metabolic Pathways
This compound is involved in several metabolic pathways, including those related to the synthesis of pharmaceutical compounds. It interacts with enzymes such as acetyl-CoA carboxylase and other cofactors involved in fatty acid metabolism . These interactions can affect metabolic flux and the levels of various metabolites, influencing overall cellular metabolism .
Transport and Distribution
Within cells and tissues, this compound is transported and distributed through various mechanisms. It can interact with transporters and binding proteins that facilitate its movement across cellular membranes . The localization and accumulation of this compound within specific tissues can affect its activity and function, with higher concentrations often observed in target tissues such as the brain and liver .
Subcellular Localization
The subcellular localization of this compound is crucial for its activity and function. It is often directed to specific compartments or organelles through targeting signals or post-translational modifications . For example, this compound may be localized to the mitochondria, where it can influence metabolic processes and energy production . The precise localization within cells can determine the specific biochemical pathways that this compound affects .
Preparation Methods
Synthetic Routes and Reaction Conditions: Acetylurea can be synthesized through several methods. One common method involves the reaction of urea with an acylating reagent such as acetic anhydride or acetyl chloride. The reaction typically proceeds as follows: [ \text{Urea} + \text{Acetic Anhydride} \rightarrow \text{this compound} + \text{Acetic Acid} ]
Industrial Production Methods: In industrial settings, this compound is often produced by reacting urea with acetic anhydride under controlled conditions. The reaction is typically carried out in a solvent such as water or an organic solvent, and the product is purified through crystallization or other separation techniques .
Chemical Reactions Analysis
Types of Reactions: Acetylurea undergoes various chemical reactions, including:
Hydrolysis: this compound can be hydrolyzed to produce urea and acetic acid.
Oxidation: It can be oxidized to form different products depending on the oxidizing agent used.
Substitution: this compound can participate in substitution reactions where the acetyl group is replaced by other functional groups.
Common Reagents and Conditions:
Hydrolysis: Typically carried out in the presence of water and an acid or base catalyst.
Oxidation: Common oxidizing agents include potassium permanganate and hydrogen peroxide.
Substitution: Various reagents can be used depending on the desired substitution product.
Major Products Formed:
Hydrolysis: Urea and acetic acid.
Oxidation: Products vary based on the oxidizing agent.
Substitution: Products depend on the substituent introduced.
Scientific Research Applications
Acetylurea has several applications in scientific research:
Chemistry: Used as a reagent in organic synthesis and as a building block for more complex molecules.
Biology: Studied for its potential biological activities, including anticonvulsant properties.
Medicine: Investigated for its potential use in pharmaceuticals, particularly in the development of anticonvulsant drugs.
Industry: Used in the production of various chemicals and as an intermediate in chemical manufacturing
Comparison with Similar Compounds
Acetylurea is similar to other urea derivatives, such as phenylthis compound and ethylphenacemide. it is unique in its specific acetyl group substitution, which imparts distinct chemical and biological properties. Compared to phenylthis compound, this compound has a simpler structure and different pharmacological effects. Similar compounds include:
- Phenylthis compound
- Ethylphenacemide
- Chlorphenacemide
- Acetylpheneturide .
This compound’s unique properties make it a valuable compound in various fields of research and industry.
Properties
IUPAC Name |
N-carbamoylacetamide | |
---|---|---|
Source | PubChem | |
URL | https://pubchem.ncbi.nlm.nih.gov | |
Description | Data deposited in or computed by PubChem | |
InChI |
InChI=1S/C3H6N2O2/c1-2(6)5-3(4)7/h1H3,(H3,4,5,6,7) | |
Source | PubChem | |
URL | https://pubchem.ncbi.nlm.nih.gov | |
Description | Data deposited in or computed by PubChem | |
InChI Key |
GKRZNOGGALENQJ-UHFFFAOYSA-N | |
Source | PubChem | |
URL | https://pubchem.ncbi.nlm.nih.gov | |
Description | Data deposited in or computed by PubChem | |
Canonical SMILES |
CC(=O)NC(=O)N | |
Source | PubChem | |
URL | https://pubchem.ncbi.nlm.nih.gov | |
Description | Data deposited in or computed by PubChem | |
Molecular Formula |
C3H6N2O2 | |
Source | PubChem | |
URL | https://pubchem.ncbi.nlm.nih.gov | |
Description | Data deposited in or computed by PubChem | |
DSSTOX Substance ID |
DTXSID9060445 | |
Record name | Acetamide, N-(aminocarbonyl)- | |
Source | EPA DSSTox | |
URL | https://comptox.epa.gov/dashboard/DTXSID9060445 | |
Description | DSSTox provides a high quality public chemistry resource for supporting improved predictive toxicology. | |
Molecular Weight |
102.09 g/mol | |
Source | PubChem | |
URL | https://pubchem.ncbi.nlm.nih.gov | |
Description | Data deposited in or computed by PubChem | |
CAS No. |
591-07-1 | |
Record name | Acetylurea | |
Source | CAS Common Chemistry | |
URL | https://commonchemistry.cas.org/detail?cas_rn=591-07-1 | |
Description | CAS Common Chemistry is an open community resource for accessing chemical information. Nearly 500,000 chemical substances from CAS REGISTRY cover areas of community interest, including common and frequently regulated chemicals, and those relevant to high school and undergraduate chemistry classes. This chemical information, curated by our expert scientists, is provided in alignment with our mission as a division of the American Chemical Society. | |
Explanation | The data from CAS Common Chemistry is provided under a CC-BY-NC 4.0 license, unless otherwise stated. | |
Record name | Acetylurea | |
Source | ChemIDplus | |
URL | https://pubchem.ncbi.nlm.nih.gov/substance/?source=chemidplus&sourceid=0000591071 | |
Description | ChemIDplus is a free, web search system that provides access to the structure and nomenclature authority files used for the identification of chemical substances cited in National Library of Medicine (NLM) databases, including the TOXNET system. | |
Record name | Acetylurea | |
Source | DTP/NCI | |
URL | https://dtp.cancer.gov/dtpstandard/servlet/dwindex?searchtype=NSC&outputformat=html&searchlist=2766 | |
Description | The NCI Development Therapeutics Program (DTP) provides services and resources to the academic and private-sector research communities worldwide to facilitate the discovery and development of new cancer therapeutic agents. | |
Explanation | Unless otherwise indicated, all text within NCI products is free of copyright and may be reused without our permission. Credit the National Cancer Institute as the source. | |
Record name | Acetamide, N-(aminocarbonyl)- | |
Source | EPA Chemicals under the TSCA | |
URL | https://www.epa.gov/chemicals-under-tsca | |
Description | EPA Chemicals under the Toxic Substances Control Act (TSCA) collection contains information on chemicals and their regulations under TSCA, including non-confidential content from the TSCA Chemical Substance Inventory and Chemical Data Reporting. | |
Record name | Acetamide, N-(aminocarbonyl)- | |
Source | EPA DSSTox | |
URL | https://comptox.epa.gov/dashboard/DTXSID9060445 | |
Description | DSSTox provides a high quality public chemistry resource for supporting improved predictive toxicology. | |
Record name | Acetylurea | |
Source | European Chemicals Agency (ECHA) | |
URL | https://echa.europa.eu/substance-information/-/substanceinfo/100.008.818 | |
Description | The European Chemicals Agency (ECHA) is an agency of the European Union which is the driving force among regulatory authorities in implementing the EU's groundbreaking chemicals legislation for the benefit of human health and the environment as well as for innovation and competitiveness. | |
Explanation | Use of the information, documents and data from the ECHA website is subject to the terms and conditions of this Legal Notice, and subject to other binding limitations provided for under applicable law, the information, documents and data made available on the ECHA website may be reproduced, distributed and/or used, totally or in part, for non-commercial purposes provided that ECHA is acknowledged as the source: "Source: European Chemicals Agency, http://echa.europa.eu/". Such acknowledgement must be included in each copy of the material. ECHA permits and encourages organisations and individuals to create links to the ECHA website under the following cumulative conditions: Links can only be made to webpages that provide a link to the Legal Notice page. | |
Record name | ACETYLUREA | |
Source | FDA Global Substance Registration System (GSRS) | |
URL | https://gsrs.ncats.nih.gov/ginas/app/beta/substances/8KRU31I919 | |
Description | The FDA Global Substance Registration System (GSRS) enables the efficient and accurate exchange of information on what substances are in regulated products. Instead of relying on names, which vary across regulatory domains, countries, and regions, the GSRS knowledge base makes it possible for substances to be defined by standardized, scientific descriptions. | |
Explanation | Unless otherwise noted, the contents of the FDA website (www.fda.gov), both text and graphics, are not copyrighted. They are in the public domain and may be republished, reprinted and otherwise used freely by anyone without the need to obtain permission from FDA. Credit to the U.S. Food and Drug Administration as the source is appreciated but not required. | |
Retrosynthesis Analysis
AI-Powered Synthesis Planning: Our tool employs the Template_relevance Pistachio, Template_relevance Bkms_metabolic, Template_relevance Pistachio_ringbreaker, Template_relevance Reaxys, Template_relevance Reaxys_biocatalysis model, leveraging a vast database of chemical reactions to predict feasible synthetic routes.
One-Step Synthesis Focus: Specifically designed for one-step synthesis, it provides concise and direct routes for your target compounds, streamlining the synthesis process.
Accurate Predictions: Utilizing the extensive PISTACHIO, BKMS_METABOLIC, PISTACHIO_RINGBREAKER, REAXYS, REAXYS_BIOCATALYSIS database, our tool offers high-accuracy predictions, reflecting the latest in chemical research and data.
Strategy Settings
Precursor scoring | Relevance Heuristic |
---|---|
Min. plausibility | 0.01 |
Model | Template_relevance |
Template Set | Pistachio/Bkms_metabolic/Pistachio_ringbreaker/Reaxys/Reaxys_biocatalysis |
Top-N result to add to graph | 6 |
Feasible Synthetic Routes
ANone: The molecular weight of AU is 102.09 g/mol.
ANone: Yes, studies have utilized various spectroscopic techniques to characterize AU, including:
- Nuclear Magnetic Resonance (NMR) Spectroscopy: 1H, 13C, and 31P NMR have been used to confirm the structure of AU and its derivatives. []
- Infrared (IR) Spectroscopy: IR spectroscopy helps identify functional groups present in AU, such as carbonyl and amine groups. []
- Mass Spectrometry (MS): MS techniques can determine the molecular weight and fragmentation pattern of AU, providing structural information. []
- Nuclear Quadrupole Resonance (NQR): 35Cl NQR has been employed to investigate the structure of chlorinated AU derivatives. []
ANone: Current research primarily focuses on AU as a synthetic building block or a metabolite, with limited exploration of its catalytic applications. Further studies are required to explore its potential as a catalyst.
ANone: Yes, computational chemistry plays a role in understanding AU and its derivatives.
- Hückel Molecular Orbital (HMO) calculations: HMO calculations, employing Hess-Schaad heteroatom parameters, have been used to determine bond-contribution values for the total π-bonding energies of acyclic AU derivatives. These calculations revealed the absence of resonance stabilization in molecules with six-, eight-, and ten-membered rings composed of CO-N linkages. []
ANone: Research on AU derivatives, particularly in the context of anticonvulsant activity, reveals a strong structure-activity relationship.
- Ring opening and decarboxylation: Conversion of barbiturates like phenobarbital and barbital (which contain a cyclic ureide moiety) to their corresponding dialkyl acetylurea analogs (e.g., ethylphenylthis compound and diethylthis compound) through ring opening and decarboxylation leads to a significant reduction in liver tumor-promoting activity. []
- Substitution at the phenyl ring: Studies comparing the anticonvulsant activity of phenylhydantoins and their corresponding phenylacetylureas indicate that substitution on the phenyl ring influences potency. [] For instance, the presence of an ethyl group in ethylphenacemide, compared to phenacemide, alters its conformational properties and potentially contributes to its increased anticonvulsant activity. []
- Presence of the this compound moiety: The this compound moiety appears crucial for anticonvulsant activity. Both phenacemide and ethylphenacemide, despite being straight-chain this compound compounds, adopt a pseudocyclic conformation in their crystalline state due to intramolecular hydrogen bonding between the amide and carbonyl groups. This conformational similarity to diphenylhydantoin, another anticonvulsant, suggests a shared mechanism of action involving the spatial arrangement of specific functional groups. []
- Hydrolysis: 2,6-Dichloro-1,3,4-diazaphosphorine, a compound derived from AU, is stable during vacuum distillation but undergoes hydrolysis in air. []
- Stability during synthesis: AU derivatives, like N-acetylureido-α-5-trifluoromethylpropionamide, can be synthesized with reasonable stability. []
A: Studies using 14C and 15N-labeled acetamide and AU in sheep have provided insights into AU metabolism. []
- Post-ruminal degradation: AU is metabolized outside the rumen, as evidenced by the appearance of 15N-labeled urea (from the labeled AU) in the rumen via rumino-hepatic circulation. This 15N-urea then participates in bacterial protein synthesis. []
ANone: While detailed pharmacodynamic studies on AU itself are limited, research on its derivative, pheneturide (ethylphenacemide), provides insights into potential mechanisms:
- Anticonvulsant activity: Pheneturide is effective in treating various forms of myoclonus, a neurological disorder characterized by involuntary muscle jerks. [] Its effectiveness suggests that AU derivatives might exert their effects through modulating neuronal excitability. [, ]
ANone: Research highlights the following:
- Anticonvulsant activity: Pheneturide, an AU derivative, exhibits efficacy in controlling myoclonus in a significant number of patients. []
ANone: Currently, there's limited data available on specific resistance mechanisms to AU or its derivatives. Further research is needed in this area.
Disclaimer and Information on In-Vitro Research Products
Please be aware that all articles and product information presented on BenchChem are intended solely for informational purposes. The products available for purchase on BenchChem are specifically designed for in-vitro studies, which are conducted outside of living organisms. In-vitro studies, derived from the Latin term "in glass," involve experiments performed in controlled laboratory settings using cells or tissues. It is important to note that these products are not categorized as medicines or drugs, and they have not received approval from the FDA for the prevention, treatment, or cure of any medical condition, ailment, or disease. We must emphasize that any form of bodily introduction of these products into humans or animals is strictly prohibited by law. It is essential to adhere to these guidelines to ensure compliance with legal and ethical standards in research and experimentation.