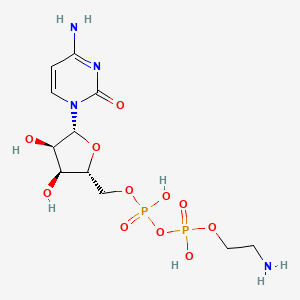
CDP-ethanolamine
Overview
Description
Mechanism of Action
Target of Action
CDP-ethanolamine, also known as CDP ethanolamine, primarily targets the biosynthesis of phosphatidylethanolamine (PE) . PE is a major inner-membrane lipid in the plasma and mitochondrial membranes . It plays a particularly important role in the inner leaflet of these membranes .
Mode of Action
This compound interacts with its targets through the This compound pathway , often referred to as the Kennedy pathway . This pathway involves the conversion of ethanolamine (Etn) and diacylglycerol (DAG) or alkyl-acyl-glycerol (AAG) to PE through three metabolic steps . The first step involves the conversion of Etn to phosphoethanolamine using ATP, leaving ADP as a by-product .
Biochemical Pathways
The this compound pathway is the sole pathway for the de novo synthesis of PE . This pathway is crucial for maintaining proper phospholipid homeostasis when major biosynthetic pathways are impaired . It is worth noting that the this compound pathway is distinct from the CDP-choline pathway and the phosphatidylserine decarboxylation pathway .
Pharmacokinetics
It is known that this compound is involved in the metabolism of the two main lipids in eukaryotic cell membranes .
Result of Action
The action of this compound results in the production of PE, which makes up 15–25% of the total lipids in the cell . PE plays a crucial role in inducing negative curvature when incorporated in cell membranes, contributing to the processes of cell fusion and fission . It is also important for the maintenance of cristae in the inner mitochondrial membrane, which increases surface area and maximizes ATP production .
Biochemical Analysis
Biochemical Properties
CDP-ethanolamine is involved in the biosynthesis of phosphatidylethanolamine through the Kennedy pathway. This pathway consists of three enzymatic steps: the phosphorylation of ethanolamine by ethanolamine kinase, the conversion of phosphoethanolamine to this compound by CTP:phosphoethanolamine cytidylyltransferase, and the final transfer of the ethanolamine phosphate group to diacylglycerol by ethanolamine phosphotransferase . This compound interacts with several enzymes, including ethanolamine kinase, CTP:phosphoethanolamine cytidylyltransferase, and ethanolamine phosphotransferase, to facilitate the synthesis of phosphatidylethanolamine .
Cellular Effects
This compound influences various cellular processes, including membrane biogenesis, cell signaling, and metabolism. It is essential for maintaining the structural integrity of cell membranes and plays a role in membrane fusion, lipid droplet formation, and autophagosome formation . Additionally, this compound affects gene expression and cellular metabolism by modulating the levels of phosphatidylethanolamine, which is involved in various signaling pathways . For example, the this compound pathway regulates skeletal muscle diacylglycerol content and mitochondrial biogenesis without altering insulin sensitivity .
Molecular Mechanism
At the molecular level, this compound exerts its effects through its involvement in the Kennedy pathway. The compound binds to CTP:phosphoethanolamine cytidylyltransferase, facilitating the conversion of phosphoethanolamine to this compound . This interaction is crucial for the subsequent transfer of the ethanolamine phosphate group to diacylglycerol by ethanolamine phosphotransferase, leading to the synthesis of phosphatidylethanolamine . The molecular mechanism of this compound also involves its role in enzyme activation and regulation of gene expression related to phospholipid metabolism .
Temporal Effects in Laboratory Settings
In laboratory settings, the effects of this compound can vary over time. The stability and degradation of this compound are critical factors influencing its long-term effects on cellular function . Studies have shown that the compound remains stable under specific conditions, but its degradation can lead to alterations in phosphatidylethanolamine levels and subsequent cellular effects . Long-term exposure to this compound has been observed to impact cellular processes such as membrane integrity and lipid metabolism .
Dosage Effects in Animal Models
The effects of this compound vary with different dosages in animal models. Studies have demonstrated that low to moderate doses of this compound can enhance phosphatidylethanolamine synthesis and improve cellular function . High doses may lead to toxic effects, including disruptions in lipid metabolism and cellular signaling pathways . It is essential to determine the optimal dosage to maximize the beneficial effects of this compound while minimizing potential adverse effects .
Metabolic Pathways
This compound is a key intermediate in the Kennedy pathway, which is responsible for the de novo synthesis of phosphatidylethanolamine . This pathway involves the conversion of ethanolamine to phosphoethanolamine by ethanolamine kinase, followed by the formation of this compound by CTP:phosphoethanolamine cytidylyltransferase, and the final synthesis of phosphatidylethanolamine by ethanolamine phosphotransferase . This compound also interacts with other metabolic pathways, including those involved in glycerophospholipid metabolism and ether lipid metabolism .
Transport and Distribution
This compound is transported and distributed within cells and tissues through various mechanisms. It is synthesized in the endoplasmic reticulum and transported to other cellular compartments, including the Golgi apparatus and mitochondria . Lipid transport proteins play a crucial role in the distribution of this compound and its incorporation into phosphatidylethanolamine . The localization and accumulation of this compound within specific cellular compartments are essential for its function in phospholipid biosynthesis .
Subcellular Localization
The subcellular localization of this compound is primarily in the endoplasmic reticulum, where it is synthesized and utilized for phosphatidylethanolamine production . This compound is also found in the Golgi apparatus and mitochondria, where it contributes to the synthesis and maintenance of phospholipid homeostasis . The targeting signals and post-translational modifications of enzymes involved in the Kennedy pathway play a crucial role in directing this compound to specific cellular compartments .
Preparation Methods
Synthetic Routes and Reaction Conditions: CDP-ethanolamine is synthesized through the this compound pathway, also known as the Kennedy pathway. This pathway involves three enzymatic steps:
Phosphorylation of Ethanolamine: Ethanolamine is phosphorylated by ethanolamine kinase to form phosphoethanolamine.
Formation of this compound: Phosphoethanolamine reacts with cytidine triphosphate (CTP) in the presence of ethanolamine-phosphate cytidylyltransferase to form this compound.
Formation of Phosphatidylethanolamine: this compound reacts with diacylglycerol (DAG) to form phosphatidylethanolamine, catalyzed by ethanolaminephosphotransferase.
Industrial Production Methods: In industrial settings, the production of this compound involves the use of recombinant enzymes to catalyze the reactions efficiently. The process typically requires optimized conditions such as specific pH, temperature, and the presence of cofactors like magnesium ions to enhance enzyme activity .
Chemical Reactions Analysis
Types of Reactions: CDP-ethanolamine primarily undergoes substitution reactions. The most notable reaction is its conversion to phosphatidylethanolamine through the displacement of cytidine monophosphate (CMP) by diacylglycerol .
Common Reagents and Conditions:
Ethanolamine kinase: Catalyzes the phosphorylation of ethanolamine.
Ethanolamine-phosphate cytidylyltransferase: Facilitates the formation of this compound from phosphoethanolamine and CTP.
Ethanolaminephosphotransferase: Catalyzes the final step, forming phosphatidylethanolamine from this compound and diacylglycerol.
Major Products:
Phosphatidylethanolamine: The primary product formed from the reaction of this compound with diacylglycerol.
Scientific Research Applications
CDP-ethanolamine has a wide range of applications in scientific research:
Chemistry: It is used in the study of lipid biosynthesis and membrane dynamics.
Industry: It is used in the production of liposomes and other lipid-based delivery systems for pharmaceuticals.
Comparison with Similar Compounds
CDP-Choline: Involved in the biosynthesis of phosphatidylcholine, another major phospholipid in cellular membranes.
CDP-Glycerol: Participates in the biosynthesis of teichoic acids in bacterial cell walls.
Uniqueness: CDP-ethanolamine is unique due to its specific involvement in the synthesis of phosphatidylethanolamine, which is crucial for maintaining the structural integrity and functionality of mitochondrial and plasma membranes .
Properties
IUPAC Name |
[2-aminoethoxy(hydroxy)phosphoryl] [(2R,3S,4R,5R)-5-(4-amino-2-oxopyrimidin-1-yl)-3,4-dihydroxyoxolan-2-yl]methyl hydrogen phosphate | |
---|---|---|
Source | PubChem | |
URL | https://pubchem.ncbi.nlm.nih.gov | |
Description | Data deposited in or computed by PubChem | |
InChI |
InChI=1S/C11H20N4O11P2/c12-2-4-23-27(19,20)26-28(21,22)24-5-6-8(16)9(17)10(25-6)15-3-1-7(13)14-11(15)18/h1,3,6,8-10,16-17H,2,4-5,12H2,(H,19,20)(H,21,22)(H2,13,14,18)/t6-,8-,9-,10-/m1/s1 | |
Source | PubChem | |
URL | https://pubchem.ncbi.nlm.nih.gov | |
Description | Data deposited in or computed by PubChem | |
InChI Key |
WVIMUEUQJFPNDK-PEBGCTIMSA-N | |
Source | PubChem | |
URL | https://pubchem.ncbi.nlm.nih.gov | |
Description | Data deposited in or computed by PubChem | |
Canonical SMILES |
C1=CN(C(=O)N=C1N)C2C(C(C(O2)COP(=O)(O)OP(=O)(O)OCCN)O)O | |
Source | PubChem | |
URL | https://pubchem.ncbi.nlm.nih.gov | |
Description | Data deposited in or computed by PubChem | |
Isomeric SMILES |
C1=CN(C(=O)N=C1N)[C@H]2[C@@H]([C@@H]([C@H](O2)COP(=O)(O)OP(=O)(O)OCCN)O)O | |
Source | PubChem | |
URL | https://pubchem.ncbi.nlm.nih.gov | |
Description | Data deposited in or computed by PubChem | |
Molecular Formula |
C11H20N4O11P2 | |
Source | PubChem | |
URL | https://pubchem.ncbi.nlm.nih.gov | |
Description | Data deposited in or computed by PubChem | |
Molecular Weight |
446.24 g/mol | |
Source | PubChem | |
URL | https://pubchem.ncbi.nlm.nih.gov | |
Description | Data deposited in or computed by PubChem | |
Physical Description |
Solid | |
Record name | CDP-ethanolamine | |
Source | Human Metabolome Database (HMDB) | |
URL | http://www.hmdb.ca/metabolites/HMDB0001564 | |
Description | The Human Metabolome Database (HMDB) is a freely available electronic database containing detailed information about small molecule metabolites found in the human body. | |
Explanation | HMDB is offered to the public as a freely available resource. Use and re-distribution of the data, in whole or in part, for commercial purposes requires explicit permission of the authors and explicit acknowledgment of the source material (HMDB) and the original publication (see the HMDB citing page). We ask that users who download significant portions of the database cite the HMDB paper in any resulting publications. | |
CAS No. |
3036-18-8 | |
Record name | CDP-ethanolamine | |
Source | CAS Common Chemistry | |
URL | https://commonchemistry.cas.org/detail?cas_rn=3036-18-8 | |
Description | CAS Common Chemistry is an open community resource for accessing chemical information. Nearly 500,000 chemical substances from CAS REGISTRY cover areas of community interest, including common and frequently regulated chemicals, and those relevant to high school and undergraduate chemistry classes. This chemical information, curated by our expert scientists, is provided in alignment with our mission as a division of the American Chemical Society. | |
Explanation | The data from CAS Common Chemistry is provided under a CC-BY-NC 4.0 license, unless otherwise stated. | |
Record name | CDP ethanolamine | |
Source | ChemIDplus | |
URL | https://pubchem.ncbi.nlm.nih.gov/substance/?source=chemidplus&sourceid=0003036188 | |
Description | ChemIDplus is a free, web search system that provides access to the structure and nomenclature authority files used for the identification of chemical substances cited in National Library of Medicine (NLM) databases, including the TOXNET system. | |
Record name | CDP-ethanolamine | |
Source | Human Metabolome Database (HMDB) | |
URL | http://www.hmdb.ca/metabolites/HMDB0001564 | |
Description | The Human Metabolome Database (HMDB) is a freely available electronic database containing detailed information about small molecule metabolites found in the human body. | |
Explanation | HMDB is offered to the public as a freely available resource. Use and re-distribution of the data, in whole or in part, for commercial purposes requires explicit permission of the authors and explicit acknowledgment of the source material (HMDB) and the original publication (see the HMDB citing page). We ask that users who download significant portions of the database cite the HMDB paper in any resulting publications. | |
Retrosynthesis Analysis
AI-Powered Synthesis Planning: Our tool employs the Template_relevance Pistachio, Template_relevance Bkms_metabolic, Template_relevance Pistachio_ringbreaker, Template_relevance Reaxys, Template_relevance Reaxys_biocatalysis model, leveraging a vast database of chemical reactions to predict feasible synthetic routes.
One-Step Synthesis Focus: Specifically designed for one-step synthesis, it provides concise and direct routes for your target compounds, streamlining the synthesis process.
Accurate Predictions: Utilizing the extensive PISTACHIO, BKMS_METABOLIC, PISTACHIO_RINGBREAKER, REAXYS, REAXYS_BIOCATALYSIS database, our tool offers high-accuracy predictions, reflecting the latest in chemical research and data.
Strategy Settings
Precursor scoring | Relevance Heuristic |
---|---|
Min. plausibility | 0.01 |
Model | Template_relevance |
Template Set | Pistachio/Bkms_metabolic/Pistachio_ringbreaker/Reaxys/Reaxys_biocatalysis |
Top-N result to add to graph | 6 |
Feasible Synthetic Routes
Disclaimer and Information on In-Vitro Research Products
Please be aware that all articles and product information presented on BenchChem are intended solely for informational purposes. The products available for purchase on BenchChem are specifically designed for in-vitro studies, which are conducted outside of living organisms. In-vitro studies, derived from the Latin term "in glass," involve experiments performed in controlled laboratory settings using cells or tissues. It is important to note that these products are not categorized as medicines or drugs, and they have not received approval from the FDA for the prevention, treatment, or cure of any medical condition, ailment, or disease. We must emphasize that any form of bodily introduction of these products into humans or animals is strictly prohibited by law. It is essential to adhere to these guidelines to ensure compliance with legal and ethical standards in research and experimentation.